.
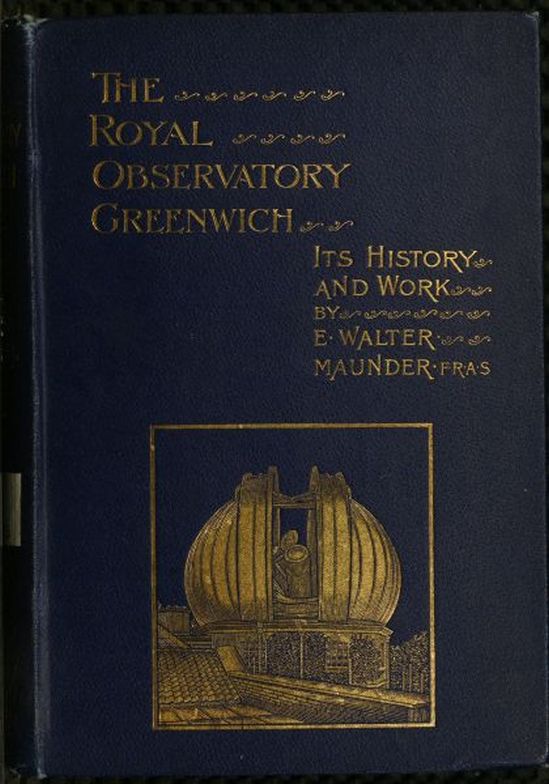
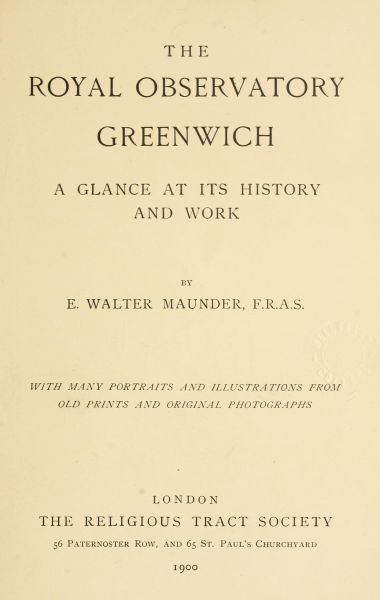
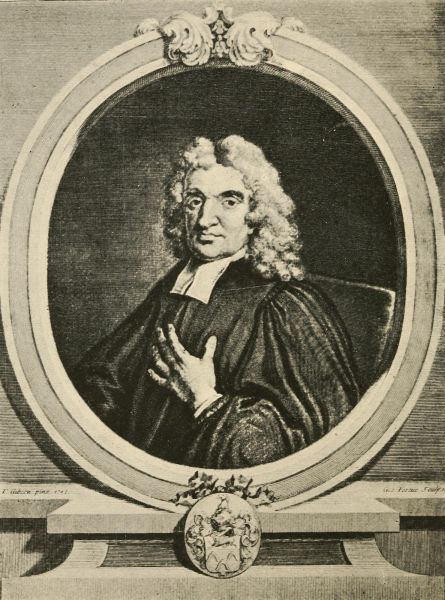
FLAMSTEED, THE FIRST ASTRONOMER ROYAL.
(From the portrait in the 'Historia Cœlestis.')
THE
ROYAL OBSERVATORY
GREENWICH
A GLANCE AT ITS HISTORY
AND WORK
BY
E. WALTER MAUNDER, F.R.A.S.
WITH MANY PORTRAITS AND ILLUSTRATIONS FROM
OLD PRINTS AND ORIGINAL PHOTOGRAPHS
LONDON
THE RELIGIOUS TRACT SOCIETY
56 Paternoster Row, and 65 St. Paul's Churchyard
1900
LONDON:
PRINTED BY WILLIAM CLOWES AND SONS, LIMITED,
STAMFORD STREET AND CHARING CROSS.
PREFACE
I was present on one occasion at a popular lecture delivered in Greenwich, when the lecturer referred to the way in which so many English people travel to the ends of the earth in order to see interesting or wonderful places, and yet entirely neglect places of at least equal importance in their own land. 'Ten minutes' walk from this hall,' he said, 'is Greenwich Observatory, the most famous observatory in the world. Most of you see it every day of your lives, and yet I dare say that not one in a hundred of you has ever been inside.'
Whether the lecturer was justified in the general scope of his stricture or not, the particular instance he selected was certainly unfortunate. It was not the fault of the majority of his audience that they had not entered Greenwich Observatory, since the regulations by which it is governed forbade them doing so. These rules are none too stringent, for the efficiency of the institution would certainly suffer if it were made a 'show' place, like a picture[6] gallery or museum. The work carried on therein is too continuous and important to allow of interruption by daily streams of sightseers.
To those who may at some time or other visit the Observatory it may be of interest to have at hand a short account of its history, principal instruments, and work. To the far greater number who will never be able to enter it, but who yet feel an interest in it, I would trust that this little book may prove some sort of a substitute for a personal visit.
I would wish to take this opportunity of thanking the Astronomer Royal for his kind permission to reproduce some of the astronomical photographs taken at the Observatory and to photograph the domes and instruments. I would also express my thanks to Miss Airy, for permission to reproduce the photograph of Sir G. B. Airy; to Mr. J. Nevil Maskelyne, F.R.A.S., for the portrait of Dr. Maskelyne; to Mr. Bowyer, for procuring the portraits of Bliss and Pond; to Messrs. Edney and Lacey, for many photographs of the Royal Observatory; and to the Editor of Engineering, for permission to copy two engravings of the Astrographic telescope.
E. W. M.
Royal Observatory, Greenwich,
August, 1900.
CONTENTS
CHAPTER | PAGE | |
I. | Introduction | 13 |
II. | Flamsteed | 25 |
III. | Halley and his Successors | 60 |
IV. | Airy | 102 |
V. | The Observatory Buildings | 124 |
[8]VI. | The Time Department | 146 |
VII. | The Transit and Circle Departments | 181 |
VIII. | The Altazimuth Department | 205 |
IX. | The Magnetic and Meteorological Departments | 228 |
X. | The Heliographic Department | 251 |
XI. | The Spectroscopic Department | 266 |
XII. | The Astrographic Department | 284 |
XIII. | The Double-Star Department | 303 |
Index | 317 |
LIST OF ILLUSTRATIONS
PAGE | |
Flamsteed, the First Astronomer Royal | Frontispiece |
The New Building | 7 |
General View of the Observatory Buildings from the New Dome | 12 |
Flamsteed's Sextant | 36 |
The Royal Observatory in Flamsteed's Time | 44 |
The 'Camera Stellata' in Flamsteed's Time | 52 |
Edmund Halley | 61 |
Halley's Quadrant | 69 |
James Bradley | 72 |
Graham's Zenith Sector | 77 |
Nathaniel Bliss | 83 |
Nevil Maskelyne | 87 |
Hadley's Quadrant | 91 |
John Pond | 96 |
George Biddell Airy, Astronomer Royal | 103 |
The Astronomer Royal's Room | 110 |
The South-east Tower | 115 |
W. H. M. Christie, Astronomer Royal | 121 |
The Astronomer Royal's House | 127 |
The Courtyard | 130 |
Plan of Observatory at Present Time | 134 |
The Great Clock and Porter's Lodge | 147 |
The Chronograph | 158 |
The Time-desk | 164 |
Harrison's Chronometer | 165 |
[10]The Chronometer Room | 167 |
The Chronometer Oven | 171 |
The Transit Pavilion | 174 |
'Lost in the Birkenhead' | 179 |
The Transit Circle | 189 |
The Mural Circle | 195 |
Airy's Altazimuth | 208 |
New Altazimuth Building | 211 |
The New Altazimuth | 213 |
The New Observatory as seen from Flamsteed's Observatory | 219 |
The Self-registering Thermometers | 235 |
The Anemometer Room, North-west Turret | 240 |
The Anemometer Trace | 243 |
Magnetic Pavilion—Exterior | 246 |
Magnetic Pavilion—Interior | 248 |
The Dallmeyer Photo-heliograph | 254 |
Photograph of a Group of Sun-spots | 259 |
The Great Nebula in Orion | 269 |
The Half-prism Spectroscope on the South-east Equatorial | 273 |
The Workshop | 276 |
The 30-inch Reflector with the New Spectroscope attached | 278 |
'Chart Plate' of the Pleiades | 286 |
The Control Pendulum and the Base of the Thompson Telescope | 289 |
The Astrographic Telescope | 291 |
The Driving Clock of the Astrographic Telescope | 294 |
The Thompson Telescope in the New Dome | 297 |
The Nebulæ of the Pleiades | 300 |
Double-star Observation with the South-east Equatorial | 308 |
The South-east Dome with the Shutter Open | 314 |
THE ROYAL OBSERVATORY
GREENWICH
CHAPTER I
INTRODUCTION
I had parted from a friend one day just as he met an acquaintance of his to whom I was unknown. 'Who is that?' said the newcomer, referring to me. My friend replied that I was an astronomer from Greenwich Observatory.
'Indeed; and what does he do there?'
This question completely exhausted my friend's information, for as his tastes did not lead him in the direction of astronomy, he had at no time ever concerned himself to inquire as to the nature of my official duties. 'Oh—er—why—he observes, don't you know?' and the answer, vague as it was, completely slaked the inquirer's thirst for knowledge.
It is not every one who has such exceedingly nebulous ideas of an astronomer's duties. More frequently we find that the inquirer has already formed a vivid and highly-coloured picture of the astronomer at his 'soul-entrancing work.' Resting[14] on a comfortable couch, fixed at a luxurious angle, the eye-piece of some great and perfect instrument brought most conveniently to his eye, there passes before him, in grand procession, a sight such as the winter nights, when clear and frosty, give to the ordinary gazer, but increased ten thousand times in beauty, brilliance, and wonder by the power of his telescope. For him Jupiter reveals his wind-drifted clouds and sunset colours; for him Saturn spreads his rings; for him the snows of Mars fall and melt, and a thousand lunar plains are ramparted with titanic crags; his are the star-clusters, where suns in their first warm youth swarm thicker than hiving bees; his the faint veils of nebulous smoke, the first hint of shape in worlds about to be, or, perchance, the last relics of worlds for ever dead. And beside the enjoyment of all this entrancing spectacle of celestial beauty, the fortunate astronomer sits at his telescope and discovers—always he discovers.
This, or something like it, is a very popular conception of an astronomer's experiences and duty; and consequently many, when they are told that 'discoveries' are not made at Greenwich, are inclined to consider that the Observatory has failed in its purpose. An astronomer without 'discoveries' to his record is like an angler who casts all day and comes home without fish—obviously an idle or incompetent person.
Again, it is considered that astronomy is a most transcendental science. It deals with infinite distances, with numbers beyond all power of human intellect to appreciate, and therefore it is supposed, on the[15] one hand, that it is a most elevating study, keeping the mind continually on the stretch of ecstasy, and, on the other hand, that it is utterly removed from all connection with practical, everyday, ordinary life.
These ideas as to the Royal Observatory, or ideas like them, are very widely current, and they are, in every respect, exactly and wholly wrong. First of all, Greenwich Observatory was originally founded, and has been maintained to the present day, for a strictly practical purpose. Next, instead of leading a life of dreamy ecstasy or transcendental speculation, the astronomer has, perhaps, more than any man, to give the keenest attention to minute practical details. His life, on the one side, approximates to that of the engineer; on the other, to that of the accountant. Thirdly, the professional astronomer has hardly anything to do with the show places of the sky. It is quite possible that there are many people whose sole opportunity of looking through a telescope is the penny peep through the instrument of some itinerant showman, who may have seen more of these than an active astronomer in a lifetime; while as to 'discoveries,' these lie no more within the scope of our national observatory than do geographical discoveries within that of the captain and officers of an ocean liner.
If it is not to afford the astronomer beautiful spectacles, nor to enable him to make thrilling discoveries, what is the purpose of Greenwich Observatory?
First and foremost, it is to assist navigation. The ease and certainty with which to-day thousands of miles of ocean are navigated have ceased to excite[16] any wonder. We do not even think about it. We go down to the docks and see, it may be, one steamer bound for Halifax, another for New York, a third for Charleston, a fourth for the West Indies, a fifth for Rio de Janeiro; and we unhesitatingly go on board the one bound for our chosen destination, without the faintest misgiving as to its direction. We have no more doubt about the matter than we have in choosing our train at a railway station. Yet, whilst the train is obliged to follow a narrow track already laid for it, from which it cannot swerve an inch, the steamer goes forth to traverse for many days an ocean without a single fixed mark or indication of direction; and it is exposed, moreover, to the full force of winds and currents, which may turn it from its desired path.
But for this facility of navigation, Great Britain could never have obtained her present commercial position and world-wide empire.
Part of this facility is, of course, due to the invention of the steam engine, but much less than is generally supposed. Even yet the clippers, with their roods of white canvas, are not entirely superseded; and if we could conceive of all steamships being suddenly annihilated, ere long the sailing vessels would again, as of yore, prove the
But with the art of navigation thrust back into its condition of a hundred and fifty years ago, it is doubtful whether a sufficient tide of commerce could be carried on to keep our home population supplied, or to maintain a sufficiently close political connection between these islands and our colonies.
Navigation was in a most primitive condition even as late as the middle of last century. Then the method of finding a ship's longitude at sea was the insufficient one of dead reckoning. In other words, the direction and speed of the ship were estimated as closely as possible, and so the position was carried on from day to day. The uncertainty of the method was very great, and many terrible stories might be told of the disastrous consequences which might, and often did, follow in the train of this method by guess-work. It will be sufficient, however, to cite the instance of Commodore Anson. He wanted to make the island of Juan Fernandez, where he hoped to obtain fresh water and provisions, and to recruit his crew, many of whom were suffering from that scourge of old-time navigators—scurvy. He got into its latitude easily enough, and ran eastward, believing himself to be west of the island. He was, however, really east of it, and therefore made the mainland of America. He had therefore to turn round and sail westwards, losing many days, during which the scurvy increased upon his crew, many of whom died from the terrible disease before he reached the desired island.
The necessity for finding out a ship's place when at sea had not been very keenly felt until the end[18] of the fifteenth century. It was always possible for the sailor to ascertain his latitude pretty closely, either by observing the height of the pole-star at night or the height of the sun at noonday; and so long as voyages were chiefly confined to the Mediterranean Sea, and the navigators were content for the most part to coast from point to point, rarely losing sight of land, the urgency of solving the second problem—the longitude of the ship—was not so keenly felt. But immediately the discoveries of the great Portuguese and Spanish navigators brought a wider, bolder navigation into vogue, it became a matter of the first necessity.
To take, for example, the immortal voyage of Christopher Columbus. His purpose in setting out into the west was to discover a new way to India. The Venetians and Genoese practically possessed the overland route across the Isthmus of Suez and down the Red Sea. Vasco da Gama had opened out the route eastward round the Cape. Firmly convinced that the world was a globe, Columbus saw that a third route was possible, namely, one nearly due west; and when, therefore, he reached the Bahamas, after traversing some 66° of longitude, he believed that he was in the islands of the China Sea, some 230° from Spain. Those who followed him still laboured under the same impression, and when they reached the mainland of America, believed that they were close to the shores of India, which was still distant from them by half the circumference of the globe.
Little by little the intrepid sailors of the sixteenth[19] century forced their way to a true knowledge of the size of the globe, and of the relative position of the great continents. But this knowledge was only attained after many disasters and terrible miseries; and though a new kind of navigation was established—the navigation of the open ocean, far away from any possible landmark, a navigation as different as could be conceived from the old method of coasting—yet it remained terribly risky and uncertain throughout the sixteenth century. Therefore many mathematicians endeavoured to solve the problem of determining the position of a ship when at sea. Their suggestions, however, remained entirely fruitless at the time, though in several instances they struck upon principles which are being employed at the present day.
The first country to profit by the discovery of America was Spain, and hence Spain was the first to feel keenly the pinch of the problem. In 1598, therefore, Philip III. offered a prize of 100,000 crowns to any one who would devise a method by which a captain of a vessel could determine his position when out of sight of land. Holland, which had recently started on its national existence, and which was challenging the colonial empire of Spain, followed very shortly after with the offer of a reward of 30,000 florins. Not very long after the offer of these rewards, a master mind did work out a simple method for determining the longitude, a method theoretically complete, though practically it proved inapplicable. This was Galileo, who, with his newly invented telescope, had discovered that Jupiter was attended by four satellites.
At first sight such a discovery, however interesting, would seem to have not the slightest bearing upon the sailor's craft, or upon the commercial progress of one nation or another. But Galileo quickly saw in it the promise of great practical usefulness. The question of the determination of the place of a ship when in the open ocean really resolved itself into this: How could the navigator ascertain at any time what was the true time, say at the port from which he sailed? As already pointed out, it was possible, by observing the height of the sun at noon, or of the pole-star at night, to infer the latitude of the ship. The longitude was the point of difficulty. Now, the longitude may be expressed as the difference between the local time of the place of observation and the local time at the place chosen as the standard meridian. The sailor could, indeed, obtain his own local time by observations of the height of the sun. The sun reached its greatest height at local noon, and a number of observations before and after noon would enable him to determine this with sufficient nicety.
But how was he to determine when he, perhaps, was half-way across the Atlantic, what was the local time at Genoa, Cadiz, Lisbon, Bristol, or Amsterdam, or whatever was the port from which he sailed? Galileo thought out a way by which the satellites of Jupiter could give him this information.
For as they circle round their primary, they pass in turn into its shadow, and are eclipsed by it. It needed, then, only that the satellites should be so carefully watched, that their motions, and,[21] consequently, the times of their eclipses could be foretold. It would follow, then, that if the mariner had in his almanac the local time of the standard city at which a given satellite would enter into eclipse, and he were able to note from the deck of his vessel the disappearance of the tiny point, he would ascertain the difference between the local times of the two places, or, in other words, the difference of their longitudes.
The plan was simplicity itself, but there were difficulties in carrying it out, the greatest being the impossibility of satisfactorily making telescopic observations from the moving deck of a ship at sea. Nor were the observations sufficiently sharp to be of much help. The entry of a satellite into the shadow of Jupiter is in most cases a somewhat slow process, and the moment of complete disappearance would vary according to the size of the telescope, the keenness of the observer's sight, and the transparency of the air.
As the power and commerce of Spain declined, two other nations entered into the contest for the sovereignty of the seas, and with that sovereignty predominance in the New World of America—France and England. The problem of the longitude at sea, or, as already pointed out, what amounts to the same thing, the problem how to determine when at sea the local time at some standard place, became, in consequence, of greater necessity to them.
The standard time would be easily known, if a thoroughly good chronometer which did not change its rate, and which was set to the standard time before[22] starting, was carried on board the ship. This plan had been proposed by Gemma Frisius as early as 1526, but at the time was a mere suggestion, as there were no chronometers or watches sufficiently good for the purpose. There was, however, another method of ascertaining the standard time. The moon moves pretty quickly amongst the stars, and at the present time, when its motions are well known, it is possible to draw up a table of its distances from a number of given stars at definite times for long periods in advance. This is actually done to-day in the Nautical Almanac, the moon's distance from certain stars being given for every three hours of Greenwich time. It is possible, then, by measuring these distances, and making, as in the case of the latitude, certain corrections, to find out the time at Greenwich. In short, the whole sky may be considered as a vast clock set to Greenwich time, the stars being the numbers on the dial face, and the moon the hand (for this clock has only one hand) moving amongst them.
The local apparent time—that is, the time at the place at which the ship itself was—is a simpler matter. It is noon at any place when the sun is due south—or, as we may put it a little differently, when it culminates—that is, when it reaches its highest point.
To find the longitude at sea, therefore, it was necessary to be able to predict precisely the apparent position of the moon in the sky for any time throughout the entire year, and it was also necessary that the places of the stars themselves should be very accurately known. It was therefore to gather the materials for[23] a better knowledge of the motions of the moon and the position of the stars that Greenwich Observatory was founded, whilst the Nautical Almanac was instituted to convey this information to mariners in a convenient form.
This proposal was actually made in the reign of Charles II. by a Frenchman, Le Sieur de Saint-Pierre, who, having secured an introduction to the Duchess of Portsmouth, endeavoured to obtain a reward for his scheme. It would appear that he had simply borrowed the idea from a book which an eminent French mathematician brought out forty years before, without having himself any real knowledge of the subject. But when the matter was brought before the king's notice, he desired some of the leading scientific men of the day to report upon its practicability, and the Rev. John Flamsteed was the man selected for the task. He reported that the scheme in itself was a good one, but impracticable in the then state of science. The king, who, in spite of the evil reputation which he has earned for himself, took a real interest in science, was startled when this was reported to him, and commanded the man who had drawn his attention to these deficiencies 'to apply himself,' as the king's astronomer, 'with the most exact care and diligence to the Rectifying the Tables of the Motions of the Heavens and the Places of the Fixed Stars, in order to find out the so much desired Longitude at Sea, for the perfecting the Art of Navigation.'
This man, the Rev. John Flamsteed, was accordingly appointed first Astronomer Royal at the meagre[24] salary of £100 a year, with full permission to provide himself with the instruments he might require, at his own expense. He followed out the task assigned to him with extreme devotion, amidst many difficulties and annoyances, until his death in 1719. He has been succeeded by seven Astronomers Royal, each of whom has made it his first object to carry out the original scheme of the institution; and the chief purpose of Greenwich Observatory to-day, as when it was founded in 1675, is to observe the motions of the sun, moon, and planets, and to issue accurate star catalogues.
It will be seen, therefore, that the establishment of Greenwich Observatory arose from the actual necessity of the nation. It was an essential step in its progress towards its present position as the first commercial nation. No thoughts of abstract science were in the minds of its founders; there was no desire to watch the cloud-changes on Jupiter, or to find out what Sirius was made of. The Observatory was founded for the benefit of the Royal Navy and of the general commerce of the realm; and, in essence, that which was the sole object of its foundation at the beginning has continued to be its first object down to the present time.
It was impossible that the work of the Observatory should be always confined within the above limits, and it will be my purpose, in the pages which follow, to describe when and how the chief expansions of its programme have taken place. But assistance to navigation is now, and has always been, the dominant note in its management.
CHAPTER II
FLAMSTEED
For the first century of its existence, the lives of its Astronomers Royal formed practically the history of the Royal Observatory. During this period, the Observatory was itself so small that the Astronomer Royal, with a single assistant, sufficed for the entire work. Everything, therefore, depended upon the ability, energy, and character of the actual director. There was no large organized staff, established routine, or official tradition, to keep the institution moving on certain lines, irrespective of the personal qualities of the chief. It was specially fortunate, therefore, that the first four Astronomers Royal, Flamsteed, Halley, Bradley, and Maskelyne (for Bliss, the immediate successor of Bradley, reigned for so short a time that he may be practically left out of the count), were all men of the most conspicuous ability.
It will be convenient to divide the history of the first seven Astronomers Royal into three sections. In the first, we have the founder, John Flamsteed, a pathetic and interesting figure, whom we seem to know with especial clearness, from the fulness of the memorials which he has left to us. He was succeeded by the man who was, indeed, best fitted to succeed[26] him, but whom he most hated. The second to the sixth Astronomers Royal formed what we might almost speak of as a dynasty, each in turn nominating his successor, who had entered into more or less close connection with the Observatory during the lifetime of the previous director; and the lives of these five may well form the second section. The line was interrupted after the resignation of the sixth Astronomer Royal, and the third section will be devoted to the seventh director, Airy, under whom the Observatory entered upon its modern period of expansion.
'God suffers not man to be idle, although he swim in the midst of delights; for when He had placed His own image (Adam) in a paradise so replenished (of His goodness) with varieties of all things, conducing as well to his pleasure as sustenance, that the earth produced of itself things convenient for both,—He yet (to keep him out of idleness) commands him to till, prune, and dress his pleasant, verdant habitation; and to add (if it might be) some lustre, grace, or conveniency to that place, which, as well as he, derived its original from his Creator.'
In these words John Flamsteed begins the first of several autobiographies which he has handed down to us; this particular one being written before he attained his majority, 'to keep myself from idleness and to recreate myself.'
'I was born,' he goes on, 'at Denby, in Derbyshire, in the year 1646, on the 19th day of August, at 7 hours 16 minutes after noon. My father, named Stephen, was the third son of Mr. William Flamsteed, of Little Hallam; my mother, Mary, was the daughter of Mr. John Spateman, of Derby, ironmonger. From these two I derived my beginning, whose parents were of known integrity, honesty, and[27] fortune, as they [were] of equal extraction and ingenuity; betwixt whom I [was] tenderly educated (by reason of my natural weakness, which required more than ordinary care) till I was aged three years and a fortnight; when my mother departed, leaving my father a daughter, then not a month old, with me, then weak, to his fatherly care and provision.'
The weakly, motherless boy became at an early age a voracious reader. At first, he says—
'I began to affect the volubility and ranting stories of romances; and at twelve years of age I first left off the wild ones, and betook myself to read the better sort of them, which, though they were not probable, yet carried no seeming impossibility in the fiction. Afterwards, as my reason increased, I gathered other real histories; and by the time I was fifteen years old I had read, of the ancients, Plutarch's Lives, Appian's and Tacitus's Roman Histories, Holingshed's History of the Kings of England, Davies's Life of Queen Elizabeth, Saunderson's of King Charles the First, Heyling's Geography, and many others of the moderns; besides a company of romances and other stories, of which I scarce remember a tenth at present.'
Flamsteed received his education at the free school at Derby, where he continued until the Whitsuntide of 1662, when he was nearly sixteen years of age. Two years earlier than this, however, a great misfortune fell upon him.
'At fourteen years of age,' he writes, 'when I was nearly arrived to be the head of the free-school, [I was] visited with a fit of sickness, that was followed with a consumption and other distempers, which yet did not so much hinder me in my learning, but that I still kept my station till the form broke up, and some of my fellows went to the Universities; for which, though I was designed, my father thought it not advisable to send me, by reason of my distemper.'
This was a keen disappointment to him, but seems to have really been the means of determining his career. The sickly, suffering boy could not be idle, though 'a day's short reading caused so violent a headache;' and a month or two after he had left school, he had a book lent to him—Sacrobosco's De Sphæra, in Latin—which was the beginning of his mathematical studies. A partial eclipse of the sun in September of the same year seems to have first drawn his attention to astronomical observation, and during the winter his father, who had himself a strong passion for arithmetic, instructed him in that science.
It was astonishing how quickly his appetite for his new subjects grew. The Art of Dialling, the calculation of tables of the sun's altitudes for all hours of the day, and for different latitudes, and the construction of a quadrant—'of which I was not meanly joyful'—were the occupations of this winter of illness.
In 1664 he made the acquaintanceship of two friends, Mr. George Linacre and Mr. William Litchford; the former of whom taught him to recognize many of the fixed stars, whilst the latter was the means of his introduction to a knowledge of the motions of the planets.
'I had now completed eighteen years, when the winter came on, and thrust me again into the chimney; whence the heat and dryness of the preceding summer had happily once before withdrawn me.'
The following year, 1665, was memorable to him 'for the appearance of the comet,' and for a journey[29] which he made to Ireland to be 'stroked' for his rheumatic disorder by Valentine Greatrackes, a kind of mesmerist, who had the repute of effecting wonderful cures. The journey, of which he gives a full and vivid account, occupied a month; but though he was a little better, the following winter brought him no permanent benefit.
But, ill or well, he pressed on his astronomical studies. A large partial eclipse of the sun was due the following June; he computed the particulars of it for Derby, and observed the eclipse itself to the best of his ability. He argued out for himself 'the equation of time'; the difference, that is, between time as given by the actual sun, or 'apparent time,' and that given by a perfect clock, or 'mean time.' He drew up a catalogue of seventy stars, computing their right ascensions, declinations, longitudes, and latitudes for the year 1701; he attempted to determine the inclination of the ecliptic, the mean length of the tropical year, and the actual distance of the earth from the sun. And these were the recreations of a sickly, suffering young man, not yet twenty-one years of age, and who had only begun the study of arithmetic, such as fractions and the rule of three, four years previously!
His next attempt was almanac-making, in the which he improved considerably upon those current at the time. His almanac for 1670 was rejected, however, and returned to him, and, not to lose his whole labour, he sent his calculations of an eclipse of the sun, and of five occultations of stars by the moon, which he had undertaken for the almanac, to the[30] Royal Society. He sent the paper anonymously, or, rather, signed it with an anagram, 'In mathesi a sole fundes,' for 'Johannes Flamsteedius.' His covering letter ends thus:—
'Excuse, I pray you, this juvenile heat for the concerns of science and want of better language, from one who, from the sixteenth year of his age to this instant, hath only served one bare apprenticeship in these arts, under the discouragement of friends, the want of health, and all other instructors except his better genius.'
This letter was dated November 4, 1669, and on January 14, Mr. Oldenburg, the secretary of the Society, replied to him in a letter which the young man cannot but have felt encouraging and flattering to the highest degree.
'Though you did what you could to hide your name from us,' he writes, 'yet your ingenious and useful labours for the advancement of Astronomy addressed to the noble President of the Royal Society, and some others of that illustrious body, did soon discover you to us, upon our solicitous inquiries after their worthy author.'
And after congratulating him upon his skill, and encouraging him to furnish further similar papers, he signs himself, 'Your very affectionate friend and real servant'—no unmeaning phrase, for the friendship then commenced ceased only with Oldenburg's life.
The following June, his father, pleased with the notice that some of the leading scientific men of the day were taking of his son, sent him up to London, that he might be personally acquainted with them; and he then was introduced to Sir Jonas Moore, the Surveyor of the Ordnance, who made him a present[31] of Townley's micrometer, and promised to furnish him with object-glasses for telescopes at moderate rates.
On his return journey he called at Cambridge, where he visited Dr. Barrow and Newton, and entered his name in Jesus College.
It was not until the following year, 1671, that he was enabled to complete his own observatory, as he had had to wait long for the lenses which Sir Jonas Moore and Collins had promised to procure for him. He still laboured under several difficulties, in that he had no good means for measuring time, pendulum clocks not then being common. He, therefore, with a practical good sense which was characteristic, refrained from attempting anything which lay out of his power to do well, and he devoted himself to such observations as did not require any very accurate knowledge of the time. At the same time, he was careful to ascertain the time of his observations as closely as possible, by taking the altitudes of the stars.
The next four years seem to have passed exceedingly pleasantly to him. The notes of ill-health are few. He was making rapid progress in his acquaintanceship with the work of other astronomers, particularly with those of the three marvellously gifted young men—Horrox, Crabtree, and Gascoigne—who had passed away shortly before his own birth. He was making new friends in scientific circles, and, in particular, Sir Jonas Moore was evidently esteeming him more and more highly. In 1674 he became more intimate with Newton, the occasion which led to this acquaintanceship being the amusing one, that his assistance was asked by[32] Newton, who had found himself unable to adjust a microscope, having forgotten its object-glass—not the only instance of the great mathematician's absent-mindedness.
The same year he took his degree of A.M. at Cambridge, designing to enter the Church; but Sir Jonas Moore was extremely anxious to give him official charge of an observatory, and was urging the Royal Society to build an astronomical observatory at Chelsea College, which then belonged to that body. He therefore came up to London, and resided some months with Sir Jonas Moore at the Tower. But shortly after his coming up to London, 'an accident happened,' to use his own expression, that hastened, if it did not occasion, the building of Greenwich Observatory.
'A Frenchman that called himself Le Sieur de St. Pierre, having some small skill in astronomy, and made an interest with a French lady, then in favour at Court, proposed no less than the discovery of the Longitude, and had procured a kind of Commission from the King to the Lord Brouncker, Dr. Ward (Bishop of Salisbury), Sir Christopher Wren, Sir Charles Scarborough, Sir Jonas Moore, Colonel Titus, Dr. Pell, Sir Robert Murray, Mr. Hook, and some other ingenious gentlemen about the town and Court, to receive his proposals, with power to elect, and to receive into their number, any other skilful persons; and having heard them, to give the King an account of them, with their opinion whether or no they were practicable, and would show what he pretended. Sir Jonas Moore carried me with him to one of their meetings, where I was chosen into their number; and, after, the Frenchman's proposals were read, which were:
'(1) To have the year and day of the observations.
'(2) The height of two stars, and on which side of the meridian they appeared.
'(3) The height of the moon's two limbs.
'(4) The height of the pole—all to degrees and minutes.
'It was easy to perceive, from these demands, that the sieur understood not that the best lunar tables differed from the heavens; and that, therefore, his demands were not sufficient for determining the longitude of the place where such observations were, or should be, made, from that to which the lunar tables were fitted, which I represented immediately to the company. But they, considering the interests of his patroness at Court, desired to have him furnished according to his demands. I undertook it; and having gained the moon's true place by observations made at Derby, February 23, 1672, and November 12, 1673, gave him observations such as he demanded. The half-skilled man did not think they could have been given him, and cunningly answered "They were feigned." I delivered them to Dr. Pell, February 19, 1674-5, who, returning me his answer some time after, I wrote a letter in English to the commissioners, and another in Latin to the sieur, to assure him they were not feigned, and to show them that, if they had been, yet if we had astronomical tables that would give us the two places of the fixed stars and the moon's true places, both in longitude and latitude, nearer than to half a minute, we might hope to find the longitude of places by lunar observations, but not by such as he demanded. But that we were so far from having the places of the fixed stars true, that the Tychonic Catalogues often erred ten minutes or more; that they were uncertain to three or four minutes, by reason that Tycho assumed a faulty obliquity of the ecliptic, and had employed only plain sights in his observations: and that the best lunar tables differ one-quarter, if not one-third, of a degree from the heavens; and lastly, that he might have learnt better methods than he proposed, from his countryman Morin, whom he had best consult before he made any more demands of this nature.'
This was in effect to tell St. Pierre that his proposal was neither original nor practicable. If St. Pierre had but consulted Morin's writings (Morin[34] himself had died more than eighteen years before), he would have known that practically the same proposal had been laid before Cardinal Richelieu in 1634, and had been rejected, as quite impracticable in the then state of astronomical knowledge. Possibly Flamsteed meant further to intimate that St. Pierre had simply stolen his method from Morin, hoping to trade it off upon the government of another country; in which case he would no doubt regard Flamsteed's letter as a warning that he had been found out.
Flamsteed continues:—
'I heard no more of the Frenchman after this; but was told that, my letters being shown King Charles, he startled at the assertion of the fixed stars' places being false in the catalogue; said, with some vehemence, "He must have them anew observed, examined, and corrected, for the use of his seamen;" and further (when it was urged to him how necessary it was to have a good stock of observations taken for correcting the motions of the moon and planets), with the same earnestness, "he must have it done." And when he was asked Who could, or who should do it? "The person (says he) that informs you of them." Whereupon I was appointed to it, with the incompetent allowance aforementioned; but with assurances, at the same time, of such further additions as thereafter should be found requisite for carrying on the work.'
Thus, in his twenty-ninth year, John Flamsteed became the first Astronomer Royal. In many ways he was an ideal man for the post. In the twelve years which had passed since he left school he had accomplished an amazing amount of work. Despite his constant ill-health and severe sufferings, and the circumstance—which may be inferred from many expressions in his autobiographies—that he assisted
[37] his father in his business, he had made himself master, perhaps more thoroughly than any of his contemporaries, of the entire work of a practical astronomer as it was then understood. He was an indefatigable computer; the calculation of tables of the motions of the moon and planets, which should as faithfully as possible represent their observed positions, had had an especial attraction for him, and, as has been already mentioned, some years before his appointment he had drawn up a catalogue of stars, based upon the observations of Tycho Brahe. More than that, he had not been a merely theoretical worker, he had been a practical observer of very considerable skill, and, in the dearth of suitable instruments, had already made one or two for himself, and had contemplated the making of others. In his first letter to Sir Jonas Moore he asks for instruction as to the making of object-glasses for telescopes, for he was quite prepared to set about the task of making his own. In addition to his tireless industry, which neither illness nor suffering could abate, he was a man of singularly exact and business-like habits. The precision with which he preserves and records the dates of all letters received or sent is an illustration of this. On the other hand, he had the defects of his circumstances and character. His numerous autobiographical sketches betray him, not indeed as a conceited man, in the ordinary sense of the word, but as an exceedingly self-conscious one. Devout and high-principled he most assuredly was, but, on the other hand, he shows in almost every line he wrote that he was one who could not brook anything like criticism or opposition.
Such a man, however efficient, was little likely to be happy as the first incumbent of a new and important government post; but there was another circumstance which was destined to cause him greater unhappiness still.
If we believe, as surely we must, that not only the moral and the physical progress of mankind is watched over and controlled by God's good Providence, but its intellectual progress as well, then there can be no doubt that John Flamsteed was raised up at this particular time, not merely to found Greenwich Observatory, and to assist the solution of the problem of the longitude at sea, but also, and chiefly, to become the auxiliary to a far greater mind, the journeyman to a true master-builder. But for the founding of Greenwich Observatory, and for John Flamsteed's observations made therein, the working out of Newton's grand theory of gravitation must have been hindered, and its acceptance by the men of science of his time immensely delayed. We cannot regard as accidental the combination, so fortunate for us, of Newton, the great world-genius, to work out the problem, of Flamsteed, the painstaking observer, to supply him with the materials for his work, and of the newly-founded institution, Greenwich Observatory, where Flamsteed was able to gather those materials together. This is the true debt that we owe to Flamsteed, that, little as he understood the position in which he had been placed from the standpoint from which we see it to-day, yet, to the extent of his ability, and as far as he conceived it in accordance with his duty, he gave Newton such assistance as he could.
This is how we see the matter to-day. It wore a very different aspect in Flamsteed's eyes; and the two following documents, the one, the warrant founding the Observatory and making him Astronomer Royal; the other, the warrant granting him a salary, will go far to explain his position in the matter. He had a high-sounding, official position, which could not fail to impress him with a sense of importance; whilst his salary was so insufficient that he naturally regarded himself as absolute owner of his own work.
'Warrant for the Payment of Mr. Flamsteed's Salary.
'Charles Rex.
'Whereas, we have appointed our trusty and well-beloved John Flamsteed, Master of Arts, our astronomical observator, forthwith to apply himself with the most exact care and diligence to the rectifying the tables of the motions of the heavens, and the places of the fixed stars, so as to find out the so-much-desired longitude of places for the perfecting the art of navigation, Our will and pleasure is, and we do hereby require and authorize you, for the support and maintenance of the said John Flamsteed, of whose abilities in astronomy we have very good testimony, and are well satisfied, that from time to time you pay, or cause to be paid, unto him, the said John Flamsteed, or his assigns, the yearly salary or allowance of one hundred pounds per annum; the same to be charged and borne upon the quarter-books of the Office of the Ordnance, and paid to him quarterly, by even and equal portions, by the Treasurer of our said office, the first quarter to begin and be accompted from the feast of St. Michael the Archangel last past, and so to continue during our pleasure. And for so doing, this shall be as well unto you, as to the Auditors of the Exchequer, for allowing the same, and all other our[40] officers and ministers whom it may concern, a full and sufficient warrant.
'Given at our Court at Whitehall, the 4th day of March, 1674-5.
'By his Majesty's Command,
'J. Williamson.
'To our right-trusty and well-beloved Counsellor, Sir Thomas Chichely, Knt., Master of our Ordnance, and to the Lieutenant-General of our Ordnance, and to the rest of the Officers of our Ordnance, now and for the time being, and to all and every of them.'
'Warrant for Building the Observatory.
'Charles Rex.
'Whereas, in order to the finding out of the longitude of places for perfecting navigation and astronomy, we have resolved to build a small observatory within our park at Greenwich, upon the highest ground, at or near the place where the Castle stood, with lodging-rooms for our astronomical observator and assistant, Our will and pleasure is, that according to such plot and design as shall be given you by our trusty and well-beloved Sir Christopher Wren, Knight, our surveyor-general of the place and scite of the said observatory, you cause the same to be fenced in, built and finished with all convenient speed, by such artificers and workmen as you shall appoint thereto, and that you give order unto our Treasurer of the Ordnance for the paying of such materials and workmen as shall be used and employed therein, out of such monies as shall come to your hands for old and decayed powder, which hath or shall be sold by our order of the 1st of January last, provided that the whole sum, so to be expended or paid, shall not exceed five hundred pounds; and our pleasure is, that all our officers and servants belonging to our said park be assisting to those that you shall appoint, for the doing thereof, and for so doing, this shall be to you, and to all others whom it may concern, a sufficient warrant.
'Given at our Court at Whitehall, the 22nd day of June, 1675, in the 27th year of our reign.
'By his Majesty's Command,
'J. Williamson.
'To our right-trusty and well-beloved Counsellor, Sir Thomas Chichely, Knt., Master-General of our Ordnance.'
The first question that arose, when it had been determined to found the new Observatory, was where it was to be placed. Hyde Park was suggested, and Sir Jonas Moore recommended Chelsea College, where he had already thought of establishing Flamsteed in a private observatory. Fortunately, both these localities were set aside in favour of one recommended by Sir Christopher Wren. There was a small building on the top of the hill in the Royal Park of Greenwich belonging to the Crown, and which was now of little or no use. Visible from the city, and easily accessible by that which was then the best and most convenient roadway, the river Thames, it was yet so completely out of town as to be entirely safe from the smoke of London. In Greenwich Park, too, but on the more easterly hill, Charles I. had contemplated setting up an observatory, but the pressure of events had prevented him carrying out his intention. A further practical advantage was that materials could be easily transported thither. The management of public affairs under Charles II. left much to be desired in the matter of efficiency and economy, and it was not very easy to procure what was wanted for the erection of a purely scientific building. However, the matter[42] was arranged. A gate-house demolished in the Tower supplied wood; iron, and lead, and bricks were supplied from Tilbury Fort, and these could be easily brought by water to the selected site. The sum of £500, actually £520, was further allotted from the results of a sale of spoilt gunpowder; and with these limited resources Greenwich Observatory was built.
The foundation-stone was laid August 10, 1675, and Flamsteed amused himself by drawing the horoscope of the Observatory, a fact which—in spite of his having written across the face of the horoscope Risum teneatis amici? (Can you keep from laughter, my friends?), and his having two or three years before written very severely against the imposture of astrology—has led some modern astrologers to claim him as a believer in their cult. He actually entered into residence July 10, 1676.
His position was not a bright one. The Government had, indeed, provided him with a building for his observatory, and a small house for his own residence, but he had no instrument and no assistant. The first difficulty was partly overcome for the moment by gifts or loans from Sir Jonas Moore, and by one or two small loans from the Royal Society. The death of this great friend and patron, four years after the founding of the Observatory, and only three years after his entering into residence, deprived him of several of these; it was with difficulty that he maintained against Sir Jonas' heirs his claim to the instruments which Sir Jonas had given him. There was nothing for him to do but to make his instruments himself, and in 1683 he built a mural quadrant
[45] of fifty inches radius. His circumstances improved the following year, when Lord North gave him the living of Burstow, near Horley, Surrey, Flamsteed having received ordination almost at the time of his appointment to the Astronomer Royalship. We have little or no account of the way in which he fulfilled his duties as a clergyman. Evidently he considered that his position as Astronomer Royal had the first claim upon him. At the same time, comparatively early in life he had expressed his desire to fill the clerical office, and he was a man too conscientious to neglect any duty that lay upon him. That in spite of his feeble health he often journeyed to and fro between Burstow and Greenwich we know; and we may take it as certain that at a time when the standard of clerical efficiency was extremely low, he was not one of those who
His chief source of income, however, seems to have been the private pupils whom he took in mathematics and astronomy. These numbered in the years 1676 to 1709 no fewer than 140; and as many of them were of the very first and wealthiest families in the kingdom, the gain to Flamsteed in money and influence must have been considerable. But it was most distasteful work. It was in no sense that which he felt to be his duty, and which he had at heart. It was undertaken from sheer, hard necessity, and he grudged bitterly the time and strength which it diverted from his proper calling.
How faithfully he followed that, one single circumstance will show. In the thirteen years ending 1689, he made 20,000 observations, and had revised single-handed the whole of the theories and tables of the heavenly bodies then in use.
In 1688 the death of his father brought him a considerable accession of means, and, far more important, the assistance of Abraham Sharp,[1] the first and most distinguished of the long list of Greenwich assistants, men who, though far less well known than the Astronomers Royal, have contributed scarcely less in their own field to the high reputation of the Observatory.
Sharp was not only a most careful and indefatigable calculator, he was what was even more essential for Flamsteed—a most skilful instrument-maker; and he divided for him a new mural arc of 140° and seven feet radius, with which he commenced operations on December 12, 1689. Above all, Sharp became his faithful and devoted friend and adherent, and no doubt his sympathy strengthened Flamsteed to endure the trouble which was at hand.
That trouble began in 1694, when Newton visited the Royal Observatory. At that time Flamsteed, though he had done so much, had published nothing, and Newton, who had made his discovery of the laws of gravitation some few years before, was then employed in deducing from them a complete theory of the moon's motion. This work was one of absolutely first importance. In the first place and[47] chiefly, upon the success with which it could be carried out, depended undoubtedly the acceptance of the greatest discovery which has yet been made in physical science. Secondarily—and this should, and no doubt did, appeal to Flamsteed—the perfecting of our knowledge of the movements of the moon was a primary part of the very work which he was commissioned to do as Astronomer Royal. Newton was, therefore, anxious beyond everything to receive the best possible observations of the moon's places, and he came to Flamsteed, as to the man from whom he had a right to expect to receive a supply of them. At first Flamsteed seems to have given these as fully as he was able; but it is evident that Newton chafed at the necessity for these frequent applications to Flamsteed, and to the constant need of putting pressure upon him. Flamsteed, on the other hand, as clearly evidently resented this continual demand. Feeling, as he keenly did, that, though he had been named Astronomer Royal, he had been left practically entirely without support; his instruments were entirely his own, either made or purchased by himself; his nominal salary of £100 was difficult to get, and did not nearly cover the actual current expenses of his position, he not unnaturally regarded his observations as his own exclusive property. He had a most natural dislike for his observations to be published, except after such reduction as he himself had carried through, and in the manner which he himself had chosen. The idea which was ever before him was that of carrying out a single great work that should not only be a monument to[48] his own industry and skill, but should also raise the name of England amongst scientific nations. He complained of it, therefore, both as a personal wrong and an injury to the country when some observations of Cassini's were combined with some observations of his own in order to deduce a better orbit for a comet.
Unknown to himself, therefore, he was called upon to decide a question that has proved fundamental to the policy of Greenwich Observatory, and he decided it wrongly—the question of publication. Newton had urged upon him as early as 1691 that he should not wait until he had formed an exhaustive catalogue of all the brighter stars, but that he should publish at once a catalogue of a few, which might serve as standards; but Flamsteed would not hear of it. He failed to see that his office had been created for a definite practical purpose, not for the execution of some great scheme, however important to science. All his work of thirty years had done nothing to forward navigation so long as he published nothing. But if, year by year, he had published the places of the moon and of a few standard stars, he would have advanced the art immensely and yet have not hindered himself from eventually bringing out a great catalogue. No doubt the little incident of Newton's difficulty with the microscope, of which he had forgotten the object-glass, had given Flamsteed a low opinion of Newton's qualifications as a practical astronomer. If so, he was wrong, for Newton's insight into practical matters was greater than Flamsteed's own, and his practical skill was no less, though[49] his absent-mindedness might occasionally lead him into an absurd mistake.
The following extract from Flamsteed's own 'brief History of the Observatory' gives an account of his view of Newton's action towards him in desiring the publication of his star catalogue, and at the same time it illustrates Flamsteed's touchy and suspicious nature.
'Whilst Mr. Flamsteed was busied in the laborious work of the catalogue of the fixed stars, and forced often to watch and labour by night, to fetch the materials for it from the heavens, that were to be employed by day, he often, on Sir Isaac Newton's instances, furnished him with observations of the moon's places, in order to carry on his correction of the lunar theory. A civil correspondence was carried on between them; only Mr. Flamsteed could not but take notice that as Sir Isaac was advanced in place, so he raised himself in his conversation and became more magisterial. At last, finding that Mr. Flamsteed had advanced far in his designed catalogue by the help of his country calculators, that he had made new lunar tables, and was daily advancing on the other planets, Sir Isaac Newton came to see him (Tuesday, April 11, 1704); and desiring, after dinner, to be shown in what forwardness his work was, had so much of the catalogue of the fixed stars laid before him as was then finished; together with the maps of the constellations, both those drawn by T. Weston and P. Van Somer, as also his collation of the observed places of Saturn and Jupiter, with the Rudolphine numbers. Having viewed them well, he told Mr. Flamsteed he would (i.e. he was desirous to) recommend them to the Prince privately. Mr. Flamsteed (who had long been sensible of his partiality, and heard how his two flatterers cried Sir Isaac's performances up, was sensible of the snare in the word privately) answered that would not do; and (upon Sir Isaac's demanding "why not?") that then the Prince's attendants would tell him these were but curiosities of no great use, and persuade[50] him to save that expense, that there might be the more for them to beg of him: and that the recommendation must be made publicly, to prevent any such suggestions. Sir Isaac apprehended right, that he was understood, and his designs defeated: and so took his leave not well satisfied with the refusal.
'It was November following ere Mr. Flamsteed heard from him any more: when, considering with himself that what he had done was not well understood, he set himself to examine how many folio pages his work when printed would fill; and found upon an easy computation that they would at least take up 1400. Being amazed at this, he set himself to consider them more seriously; drew up an estimate of them; and, to obviate the misrepresentations of Dr. S[loane] and some others, who had given out that what he had was inconsiderable, he delivered a copy of the estimate to Mr. Hodgson, then lately chosen a member of the Royal Society, with directions to deliver it to a friend, who he knew would do him justice; and, on this fair account, obviate those unjust reports which had been studiously spread to his prejudice. It happened soon after, Mr. Hodgson being at a meeting, spied this person there, at the other side of the room; and therefore gave the paper to one that stood in some company betwixt them, to be handed to him. But the gentleman, mistaking his request, handed to the Secretary [Dr. Sloane], who, being a Physician, and not acquainted with astronomical terms, did not read it readily. Whereupon another in the company took it out of his hands; and, having read it distinctly, desired that the works therein mentioned might be recommended to the Prince; the charge of printing them being too great either for the author or the Royal Society. Sir Isaac closed in with this.'
The work was in consequence recommended to Prince George of Denmark, the Queen's Consort; but it was not till November 10, 1705, that the contract for the printing was signed. Two years later, the observations which he had made with
[53] his sextant in his first thirteen years of office were printed. Then came the difficulty of the catalogue. It was not complete to Flamsteed's satisfaction, and he was most unwilling to let it pass out of his hands. However, two manuscripts, comprising some three-quarters of the whole, were deposited with referees, the first of these being sealed up. The seal was broken with Flamsteed's concurrence; but the fact that it had been so broken was made by him the subject of bitter complaint later. At this critical juncture Prince George died, and a stop was put to the progress of the printing. Two years more elapsed without any advance being made, and then, in order to check any further obstruction, a committee of the Royal Society was appointed as a Board of Visitors to visit and inspect the Observatory, and so maintain a control over the Astronomer Royal. This was naturally felt by so sensitive a man as Flamsteed as a most intolerable wrong, and when he found that the printing of his catalogue had been placed in the hands of Halley as editor, a man for whom he had conceived the most violent distrust, he absolutely refused to furnish the Visitors with any further material. This led to, perhaps, the most painful scene in the lives either of Newton or Flamsteed. Flamsteed was summoned to meet the Council of the Royal Society at their rooms in Crane Court. A quorum was not present, and so the interview was not official, and no record of it is preserved in the archives. Flamsteed has himself described it with great particularity in more than one document, and it is only too easy to[54] understand the scene that took place. Newton was a man who had an absolutely morbid dread of anything like controversy, and over and over again would have preferred to have buried his choicest researches, rather than to have encountered the smallest conflict of the kind. He was perhaps, therefore, the worst man to deal with a high-principled, sensitive, and obstinate man who was in the wrong, and yet who had been so hardly dealt with that it was most natural for him to think himself wholly in the right. Flamsteed adhered absolutely to his position, from which it is clear it would have been extremely difficult for the greatest tact and consideration to have dislodged him. Newton, on his part, simply exerted his authority, and, that failing, was reduced to the miserable extremity of calling names. The scene is described by Flamsteed himself, in a letter to Abraham Sharp, as follows:—
'I have had another contest with the President[2] of the Royal Society, who had formed a plot to make my instruments theirs; and sent for me to a Committee, where only himself and two physicians (Dr. Sloane, and another as little skilful as himself) were present. The President ran himself into a great heat, and very indecent passion. I had resolved aforehand his kn—sh talk should not move me; showed him that all the instruments in the Observatory were my own; the mural arch and voluble quadrant having been made at my own charge, the rest purchased with my own money, except the sextant and two clocks, which were given me by Sir Jonas Moore, with Mr. Towneley's micrometer, his gift, some years before I came to Greenwich. This nettled him; for he has got a letter from the Secretary of State for the Royal Society[55] to be Visitors of the Observatory, and he said, "as good have no observatory as no instruments." I complained then of my catalogue being printed by Raymer, without my knowledge, and that I was robbed of the fruit of my labours. At this he fired, and called me all the ill names, puppy, etc., that he could think of. All I returned was, I put him in mind of his passion, desired him to govern it, and keep his temper: this made him rage worse, and he told me how much I had received from the Government in thirty-six years I had served. I asked what he had done for the £500 per annum that he had received ever since he had settled in London. This made him calmer; but finding him going to burst out again, I only told him my catalogue, half finished, was delivered into his hands, on his own request, sealed up. He could not deny it, but said Dr. Arbuthnott had procured the Queen's order for opening it. This, I am persuaded, was false; or it was got after it had been opened. I said nothing to him in return; but, with a little more spirit than I had hitherto showed, told them that God (who was seldom spoken of with due reverence in that meeting) had hitherto prospered all my labours, and I doubted not would do so to a happy conclusion; took my leave and left them. Dr. Sloane had said nothing all this while; the other Doctor told me I was proud, and insulted the President, and ran into the same passion with the President. At my going out, I called to Dr. Sloane, told him he had behaved himself civilly, and thanked him for it. I saw Raymer after, drank a dish of coffee with him, and told him, still calmly, of the villany of his conduct, and called it blockish. Since then they let me be quiet; but how long they will do so I know not, nor am I solicitous.'
The Visitors continued the printing, Halley being the editor, and the work appeared in 1712 under the title of Historia Cœlestis. This seemed to Flamsteed the greatest wrong of all. The work as it appeared seemed to him so full of errors, wilfully or accidentally inserted, as to be the greatest blot upon his fair[56] fame, and he set himself, though now an old man, to work it out de novo and at his own expense. To that purpose he devoted the remaining seven years of his life. Few things can be more pathetic than the letters which he wrote in that period referring to it. He was subject to the attacks of one of the cruelest of all diseases—the stone; he was at all times liable to distracting headaches. He had been, from his boyhood, a great sufferer from rheumatism, and yet, in spite of all, he resolutely pushed on his self-appointed task. The following extract from one of his letters will give a more vivid idea of the brave old man than much description:—
'I can still, I praise God for it, walk from my door to the Blackheath gate and back, with a little resting at some benches I have caused to be set up betwixt them. But I found myself so tired with getting up the hill when I return from church, that at last I have bought a sedan, and am carried thither in state on Sunday mornings and back; I hope I may employ it in the afternoons, though I have not hitherto, by reason of the weather is too cold for me.'
After the death of Queen Anne, a change in the ministry enabled him to secure that three hundred copies of the total impression of four hundred of the Historia Cœlestis were handed over to him. These, except the first volume, containing his sextant observations (which had received his own approval), he burned, 'as a sacrifice to heavenly truth.' His own great work had advanced so far that the first volume was printed, and much of the second, when he himself died, on the last day of 1719. He was buried in the chancel of Burstow Church.
The completion of his work took ten years more; a work of piety and regard on the part of his assistant, Joseph Crosthwait.
When compared with the catalogues that have gone before, it was a work of wonderful accuracy. Nevertheless, as Caroline Herschel showed, nearly a century later, not a few errors had crept into it. Some of the stars are non-existent, others have been catalogued in more than one constellation; important stars have been altogether omitted. Perhaps the most serious fault arises from the neglect of Flamsteed to accept from Newton a practical hint, namely, to read the barometer and thermometer at the time of his observations. Nevertheless, the work accomplished was not only wonderful under the untoward conditions in which Flamsteed was placed; it was wonderful in itself, winning from Airy the following high encomium:—
'In regard not only to accuracy of observation, and to detail in publication of the methods of observing, but also to steadiness of system followed through many years, and to completeness of calculation of the useful results deduced from the observations, this work may shame any other collection of observations in this or any other country.'
This catalogue was not Flamsteed's only achievement. He had determined the latitude of the Observatory, the obliquity of the ecliptic, and the position of the equinoctial points. He thought out an original method of obtaining the absolute right ascensions of stars by differential observations of the places of the stars and the sun near to both equinoxes. He had revised and improved Horrox's theory of the lunar[58] motions, which was by far the best existing in Flamsteed's day. He showed the existence of the long inequality of Jupiter and Saturn; that is to say, the periodic influence which they exercise upon each other. He determined the time in which the sun rotates on its axis, and the position of that axis. He observed an apparent movement of the stars in the course of a year, which he ascribed, though erroneously, to the stellar parallax, and which was explained by the third Astronomer Royal, Bradley.
Flamsteed not only met with harsh treatment during his lifetime; he has not yet received, except from a few, anything like the meed of appreciation which is his just due; but, at least, his successors in the office have not forgotten him. They have been proud that their official residence should be known as Flamsteed House, and his name is inscribed over the main entrance of the latest and finest of the Observatory buildings, and his bust looks forth from its front towards the home where he laboured so devotedly for nearly fifty years. But he has received little honour, save at Greenwich, and—in spite of the proverb—in his other home, the village of Burstow, in Surrey, of which he was for many years the rector. Here a stained glass window representing, appropriately, the Adoration of the Magi, has been recently set up to his memory, largely through the interest taken in his history by an amateur astronomer of the neighbourhood, Mr. W. Tebb, F.R.A.S.
No instrument of Flamsteed's remains in the[59] Observatory, his wife removing them after his death. But we may consider his principal instrument, the mural quadrant made for him by Abraham Sharp, as represented by the remains of a quadrant by the same artist, which was presented to the Observatory by the Rev. N. S. Heineken, in 1865, and now hangs over the door of the transit room.
CHAPTER III
HALLEY AND HIS SUCCESSORS
There is no need to give the lives of the succeeding Astronomers Royal so fully as that of Flamsteed. Not that they were inferior men to him; on the contrary, there can be little doubt that we ought to reckon some of them as his superiors, but, in the case of several, their best work was done apart from Greenwich Observatory, and before they came to it.
This was particularly the case with Edmund Halley. Born on October 29, 1656, he was ten years the junior of Flamsteed. Like Flamsteed, he came of a Derbyshire family, though he was born at Haggerston, in the parish of St. Leonard's, Shoreditch. He was educated at St. Paul's School, where he made very rapid progress, and already showed the bent of his mind. He learnt to make dials; he made himself so thoroughly acquainted with the heavens that it is said, 'If a star were displaced in the globe he would presently find it out,' and he observed the changes in the direction of the mariner's compass. In 1673 he went to Queen's College, Oxford, where he observed a sunspot in July and August, 1676, and an occultation of Mars. This was not his first astronomical observation, as, in June,
[63] 1675, he had observed an eclipse of the moon from his father's house in Winchester Street.
A much wider scheme of work than such merely casual observations now entered his mind, possibly suggested to him by Flamsteed's appointment to the direction of the new Royal Observatory. This was to make a catalogue of the southern stars. Tycho's places for the northern stars were defective enough, but there was no catalogue at all of stars below the horizon of Tycho's observatory. Here, then, was a field entirely unworked, and young Halley was so eager to enter upon it that he would not wait at Oxford to obtain his degree, but was anxious to start at once for the southern hemisphere.
His father, who was wealthy and proud of his gifted son, strongly supported him in his project. The station he selected was St. Helena, an unfortunate choice, as the skies there were almost always more or less clouded, and rain was frequent during his stay. However, he remained there a year and a half, and succeeded in making a catalogue of 341 stars. This catalogue was finally reduced by Sharp, and included in the third volume of Flamsteed's Historia Cœlestis.
In 1678 he was elected Fellow of the Royal Society, and the following year he was chosen to represent that society in a discussion with Hevelius. The question at issue was as to whether more accurate observations of the place of a star could be obtained by the use of sights without optical assistance, or by the use of a telescope. The next year he visited the Paris Observatory, and, later in the same tour, the principal cities of the Continent.
Not long after his return from this tour, Halley was led to that undertaking for which we owe him the greatest debt of gratitude, and which must be regarded as his greatest achievement.
Some fifty years before, the great Kepler had brought out the third of his well-known laws of planetary motion. These laws stated that the planets move round the sun in ellipses, of which the sun occupies one of the foci; that the straight line joining any planet with the sun moves over equal areas of space in equal periods of time; and, lastly, that the squares of the times in which the several planets complete a revolution round the sun are proportional to the cubes of their mean distances from it. These three laws were deduced from actual examination of the movements of the planets. Kepler did not work out any underlying cause of which these three laws were the consequence.
But the desire to find such an underlying cause was keen amongst astronomers, and had given rise to many researches. Amongst those at work on the subject was Halley himself. He had seen, and been able to prove, that if the planets moved in circles round the sun, with the sun in the centre, then the law of the relation between the times of revolution and the distances of the planets would show that the attractive force of the sun varied inversely as the square of the distance. The actual case, however, of motion in an ellipse was too hard for him, and he could not deal with it. Halley therefore went up to Cambridge to consult Newton, and, to his wonder and delight, found that the latter had already completely[65] solved the problem, and had proved that Kepler's three laws of planetary motion were summed up in one, namely, that the sun attracted the planets to it with a force inversely proportional to the square of the distance.
Halley was most enthusiastic over this great discovery, and he at once strongly urged Newton to publish it. Newton's unwillingness to do so was great, but at length Halley overcame his reluctance; and the Royal Society not being able at the time to afford the expense, Halley took the charges upon himself, although his own resources had been recently seriously damaged by the death of his father.
The publication of Newton's Principia, which, but for him, might never have seen the light, and most certainly would have been long delayed, is Halley's highest claim to our gratitude. But, apart from this, his record of scientific achievement is indeed a noble one. Always, from boyhood, he had taken a great interest in the behaviour of the magnetic compass, and he now followed up the study of its variations with the greatest energy. For this purpose it was necessary that he should travel, in view of the great importance of the subject to navigation. King William III. gave him a captain's commission in the Royal Navy—a curious and interesting illustration of the close connection between astronomy and the welfare of our navy—and placed him in command of a 'pink,' that is to say, a small vessel with pointed stern, named the Paramour, in which he proceeded to the southern ocean. His first voyage was unfortunate,[66] but the Paramour was recommissioned in 1699, and he sailed in it as far as south latitude 52°.
In 1701 and the succeeding year he made further voyages in the Paramour, surveying the tides and coasts of the British Channel and of the Adriatic, and helping in the fortification of Trieste. He became Savilian Professor of Geometry at Oxford in 1703, having failed twelve years previously to secure the Savilian Professorship of Astronomy, mainly through the opposition of Flamsteed, who had already formed a strong prejudice against him, which some writers have traced to Halley's detection of several errors in one of Flamsteed's tide-tables, others to Halley's supposed materialistic views. Probably the difference was innate in the two men. There was likely to be but little sympathy between the strong, masterful man of action and society and the secluded, self-conscious, suffering invalid. At any rate, in the contest between Newton and Flamsteed, which has been already described, Halley took warmly the side of the former, and was appointed to edit the publication of Flamsteed's results, and, on the death of the latter, to succeed him at the Royal Observatory.
The condition of things at Greenwich when Halley succeeded to the post of Astronomer Royal in 1720 was most discouraging. The instruments there had all belonged to Flamsteed, and therefore, most naturally, had been removed by his widow. The Observatory had practically to be begun de novo, and Halley had now almost attained the age at which in the present day an Astronomer Royal would have to retire. More fortunate, however, than[67] his predecessor, he was able to get a grant for instruments, and he equipped the Observatory as well as the resources of the time permitted, and his transit instrument and great eight-foot quadrant still hang upon the Observatory walls.
As Astronomer Royal his great work was the systematic observation of the positions of the moon through an entire saros. As is well known, a period of eighteen years and ten or eleven days brings the sun and moon very nearly into the same positions relatively to the earth which they occupied at the commencement of the period. This period was well known to the ancient Chaldeans, who gave it its name, since they had noticed that eclipses of the sun or eclipses of the moon recurred at intervals of the above length. It was Halley's desire to obtain such a set of observations of the moon through an entire saros period as to be able to deduce therefrom an improved set of tables of the moon's motion. It was an ambitious scheme for a man so much over sixty to undertake, nevertheless he carried it through successfully.
His desire to complete this scheme, and to found upon it improved lunar tables, hindered him from publishing his observations, for he feared that others might make use of them before he was in a position to complete his work himself. This omission to publish troubled Newton, who, as President of the Royal Society—the Greenwich Board of Visitors having lapsed at Queen Anne's death—drew attention at a meeting of the Royal Society, March 2, 1727, to Halley's disobedience of the order issued under Queen[68] Anne, for the prompt communication of the Observatory results. That Newton should thus have put public pressure upon Halley, the man to whom he was so much indebted, and with whom there was so close an affection, is sufficient proof that his similar attitude towards Flamsteed was one of principle and not of arbitrariness. Halley, on his side, stood firm, as Flamsteed had done, urging the danger that, by publishing before he had completed his task, he might give an opportunity to others to forestall his results. It is said—probably without sufficient ground—that this refusal broke Newton's heart and caused his death. Certainly Halley's writings in that very year show his reverence and affection for Newton to have been as keen and lively as ever.
Halley's work at the Observatory went on smoothly, on the lines he had laid down for himself, for ten years after Newton's death; but in 1737 he had a stroke of paralysis, and his health, which had been remarkably robust up to that time, began to give way. He died January 14, 1742, and was buried in the cemetery of Lee Church.
As an astronomer, his services to the science rank higher than those of his predecessor; but as Astronomer Royal, as director, that is to say, of Greenwich Observatory, he by no means accomplished as much as Flamsteed had done. Professor Grant, in his History of Physical Astronomy, says that he seems to have undervalued those habits of minute attention which are indispensable to the attainment of a high degree of excellence in the practice of astronomical observation. He was far from being sufficiently[69] careful as to the adjustment of his instruments, the going of his clocks, or the recording of his own observations. The important feature of his[70] administration was that under him the Observatory was first supplied with instruments which belonged to it.
His astronomical work apart from the Observatory was of the first importance. He practically inaugurated the study of terrestrial magnetism, and his map giving the results of his observations during his voyage in the Paramour introduced a new and most useful style of recording observations. He joined together by smooth curves places of equal variation, the result being that the chart shows at a glance, not merely the general course of the variation over the earth's surface, but its value at any spot within the limits of the chart.
Another work which has justly made his name immortal was the prediction of the return of the comet which is called by his name, to which reference will be made later. Another great scheme, and one destined to bear much fruit, was the working out of a plan to determine the distance of the sun by observations of the transit of Venus.
Of attractive appearance, pleasing manners, and ready wit, loyal, generous, and free from self-seeking, he probably was one of the most personally engaging men who ever held the office.
The salary of the Astronomer Royal remained under Halley at the same inadequate rate which it had done under Flamsteed—£100, without provision for an assistant. But in 1729 Queen Caroline, learning that Halley had actually had a captain's commission in the Royal Navy, secured for him a post-captain's pay.
Halley's work is represented at the Observatory by two of his instruments which are still preserved there, and which hang on the west wall of the present transit room: the Iron Quadrant afterwards made famous by the observations of Bradley, and 'Halley's Transit,' the first of the great series of instruments upon which the fame of Greenwich chiefly rests. This transit instrument seems to have been set up in a small room at the west end of what is now known as the North Terrace. His quadrant was mounted on the pier which is now the base of the pier of the astrographic telescope. This pier was the first extension which the Observatory received from the original building.
On the breakdown of his health Halley nominated as his successor, James Bradley; indeed, it is stated that he offered to resign in his favour. He had known him then for over twenty years, and that keen and generous appreciation of merit in others which was characteristic of Halley had led him very early to recognize Bradley's singular ability.
James Bradley was born in 1692 or 1693, of an old North of England family. His birthplace was Sherbourne, in Gloucestershire, and he was educated at North Leach Grammar School and at Baliol College, Oxford. During the years of his undergraduateship he resided much with his uncle, the Rev. James Pound, Rector of Wanstead, Essex, an ardent amateur astronomer, a frequent visitor at the Observatory in Flamsteed's time, and one of the most accurate observers in the country. From him,[74] no doubt, he derived his love of the science, and possibly some of his skill in observation.
Bradley's earliest observations seem to have been devoted to the phenomena of Jupiter's satellites and to the measures of double stars. The accuracy with which he followed up the first drew the attention of Halley, and so began a friendship which lasted through life. His observations of double stars, particularly of Castor, only just failed to show him the orbital movement of the pair, because his attention was drawn to other subjects before it had become sufficiently obvious.
In 1719 Bradley and his uncle made an attempt to determine the distance of the sun through observations of Mars when in opposition, observations which were so accurate that they sufficed to show that the distance of the sun could not be greater than 125 millions of miles, nor less than about 94 millions. The lower limit which they thus found has proved to be almost exactly correct, our best modern determinations giving it as 93 millions. The instrument with which the observations were made was a novel one, being 'moved by a machine that made it to keep pace with the stars;' in other words, it was the first, or nearly the first, example of what we should now call a clock-driven equatorial.
That same year he was offered the Vicarage of Bridstow, near Ross, in Monmouthshire, where, having by that time taken priest's orders, he was duly installed, July, 1720. To this was added the sinecure Rectory of Llandewi-Velgry; but he held both livings only a very short time. In 1721 the death of Dr.[75] John Keill rendered vacant the Savilian Professorship of Astronomy at Oxford, for which Bradley became a candidate, and was duly elected, and resigned his livings in consequence.
It was whilst he was Savilian Professor that Bradley made that great discovery which will always be associated with his name. Though professor at Oxford, he had continued to assist his uncle, Mr. Pound, at his observations at Wanstead, and after the death of the latter he still lived there as much as possible, and continued his astronomical work. But in 1725 he was invited by Mr. Samuel Molyneux, who had set up a twenty-four-foot telescope made by Graham as a zenith tube at his house on Kew Green, to verify some observations which he was making. These were of the star Gamma Draconis, a star which passes through the zenith of London, and which, therefore, had been much observed both by Flamsteed and Hooke, inasmuch as by fixing a telescope in an absolutely vertical position—a position which could be easily verified—it was easy to ascertain if there was any minute change in the apparent position of the star. Dr. Hooke had declared that there was such a change, a change due to the motion of the earth in its orbit, which would prove that the star was not an infinite distance from the earth, the seeming change of its place in the sky corresponding to the change in the place of the earth from which the observer was viewing it.
Bradley found at once that there was such a change—a marked one. It amounted to as much as 1´´ of arc in three days; but it was not in the[76] direction in which the parallax of the star would have moved it, but in the opposite. Whether, therefore, the star was near enough to show any parallax or not, some other cause was giving rise to an apparent displacement of the star, which entirely masked and overcame the effect of parallax.
So far, Bradley had but come to the same point which Flamsteed had reached. Flamsteed had detected precisely the same apparent displacement of stars, and, like Hooke, had ascribed it to parallax. Cassini had shown that this could not be the case, as the displacement was in the wrong direction; and there the matter had rested. Bradley now set to follow the question up. Other stars beside Gamma Draconis were found to show a displacement of the same general character, but the amount varied with their distance from the plane of the ecliptic, the earth's orbit. The first explanation suggested was that the axis of the earth, which moves very nearly parallel to itself as the earth moves round the sun, underwent a slight regular 'wobble' in the course of a year. To check this, a star was observed on the opposite side of the pole from Gamma Draconis; then Bradley investigated as to whether refraction might explain the difficulty, but again without success. He now was most keenly interested in the problem, and he purchased a zenith telescope of his own, made, like that of Molyneux, by Graham, and mounted it in his aunt's house at Wanstead, and observed continuously with it. The solution of the problem came at last to him as he was boating on the Thames. Watching a vane at the top of the mast,
[78] he saw with surprise that it shifted its direction every time that the boat was put about. Remarking to the boatmen that it was very odd that the wind should change just at the same moment that there was a shift in the boat's course, they replied that there was no change in the wind at all, and that the apparent change of the vane was simply due to the change of direction of the motion of the boat.
This supplied Bradley with a key to the solution of the mystery that had troubled him so long. It had been discovered long before this that light does not travel instantaneously from place to place, but takes an appreciable time to pass from one member of the solar system to another. This had been discovered by Römer from observations of the satellites of Jupiter. He had noted that the eclipses of the satellites always fell late of the computed time, when Jupiter was at his greatest distance from the earth; and Bradley's own work in the observation of those satellites had brought the fact most intimately under his own acquaintance. The result of the boating incident taught him, then, that he might look upon light as analogous to the wind blowing on the boat. As the wind, so long as it was steady, would seem to blow from one fixed quarter so long as the boat was also in rest, but as it seemed to shift its direction when the boat was moving and changed its direction, so he saw that the light coming from a particular star must seem to slightly change the direction in which it came, or, in other words, the apparent position of the star, to correspond with the movement of the earth in its orbit round the sun.
This was the celebrated discovery of the Aberration of Light, a triumph of exact observation and of clear insight. As to the exactness of Bradley's observations, it is sufficient to say that his determination of the value of the 'Constant of Aberration' gave it as 20·39´´; the value adopted to-day is 20·47´´.
On the death of Halley, in 1742, Bradley was appointed to succeed him. He found the Observatory in as utterly disheartening a condition as his predecessors had done. As already mentioned, Halley had not the same qualifications as an observer that Flamsteed had. He was, further, an old man when appointed to the post, he had no assistant provided for him, and the last five years of his life his health and strength had entirely given way. Under these circumstances, it was no wonder that Bradley found the instruments of the Observatory in a deplorable state. Nevertheless, he set to work most energetically, and in the year of his appointment he made 1500 observations in the last five months of the year. He was particularly earnest in examining the condition and the errors of his instruments; and as their defects became known to him, he was more and more anxious for a better equipment. He moved the Royal Society, therefore, to apply on his behalf for the instruments he required; and a petition from that body, in 1748, obtained what in those days must be considered the generous grant of £1000, the proceeds of the sale of old Admiralty stores. The principal instruments purchased therewith were a mural quadrant and a transit instrument, both eight feet in focal length, still preserved on the walls of the[80] transit-room. It is interesting also to note that, following in the steps of Halley, and forecasting, as it were, the magnetic observatory which Airy would found, he devoted £20 of the grant to purchasing magnetic instruments.
Meantime he had continued his observations on aberration, and had discovered that the aberration theory was not sufficient entirely to account for the apparent changes in places of stars which he had discovered. A second cause was at work, a movement of the earth's axis, a 'wobble' in its inclination, technically known as Nutation, which is due to the action of the moon, and goes through its course in a period of nineteen years.
Beside these two great discoveries of aberration and nutation, Bradley's reputation rests upon his magnificent observations of the places of more than three thousand stars. This part of his work was done with such thoroughness, that the star-places deduced from them form the basis of most of our knowledge as to the actual movements of individual stars. In particular, he was careful to investigate and to correct for the errors of his instrument, and to determine the laws of refraction, introducing corrections for changes in the readings of thermometer and barometer. His tables of refraction were used, indeed, for seventy years after his death. Of his other labours it may be sufficient to refer to his determination of the longitudes of Lisbon and of New York, and to his effort to ascertain the parallax of the sun and moon, in combination with La Caille, who was observing at the Cape of Good Hope.
As Astronomer Royal, Bradley's great achievement was the high standard to which he raised the practical work of observation. From his day onwards, also, there was always at least one assistant. His first assistant was his own nephew, John Bradley, who received the munificent salary of ten shillings a week. Still, this was not out of proportion to the then salary of the Astronomer Royal, which practically amounted only to £90. However, in 1752, Bradley was awarded a Crown pension of £250 a year. He refused the living of Greenwich, which was offered him in order to increase his emoluments, on the ground that he could not suitably fulfil the double office. Bradley's later assistants were Charles Mason and Charles Green.
Bradley's last work was the preparation for the observations of the transit of Venus of 1761, according to the lines laid down by his predecessor, Halley. His health gave way, and he became subject to melancholia, so that the actual observations were taken by the Rev. Nathaniel Bliss, who succeeded him in his office after his death, in 1762. He was buried at Minchinhampton.
So far as we know Bradley's character, he seems to have been a gentle, modest, unassuming man, entirely free from self-seeking, and indifferent to personal gain. He was in many ways an ideal astronomer, exact, methodical, and conscientious to the last degree. His skill as an observer was his chief characteristic; and though his abilities were not equal as a mathematician or a mechanician, yet, on the one hand, he had a very clear insight into the[82] meaning of his observations, and, on the other, he was skilful enough to himself adjust, repair, and improve his instruments.
Of Bradley's instruments, there are still preserved his famous twelve-and-a-half-foot zenith sector, with which he made his two great discoveries; his brass quadrant, which in 1750 he substituted for Halley's iron quadrant; his transit instrument, and equatorial sector. Bradley added to the buildings of the Observatory that portion which is now represented by the upper and lower computing rooms, and the chronometer room, which adjoins the latter. This room—the chronometer room—was his transit room, and the position of the shutters is still marked by the window in the roof.
The Rev. Nathaniel Bliss, who succeeded Bradley, only held the office for a couple of years, and during that time was much at Oxford. He, therefore, has left no special mark behind him as Astronomer Royal.
He was born November 28, 1700. His father, like himself, Nathaniel Bliss, was a gentleman, of Bisley, Gloucestershire.
Bliss graduated at Pembroke College, Oxford, as B.A. in 1720, and M.A. in 1723. He became the Rector of St. Ebb's, Oxford, in 1736, and on Halley's death succeeded him as Savilian Professor of Geometry. He supplied Bradley with his observations of Jupiter's satellites, and from time to time, at his request, rendered him some assistance at the Royal Observatory. This was particularly the case, as has
[85] been already mentioned, with respect to the transit of Venus of 1761, the observations of which were carried out by Bliss, owing to Bradley's ill-health. It was natural, therefore, that on Bradley's death he should succeed to the vacant post; but he held it too short a time to do any distinctive work. Such observations as he made seem to have been entirely in continuation of Bradley's. He took a great interest, however, in the improvement of clocks, a department in which so much was being done at this time by Graham, Ellicott, and others.
Nevil Maskelyne, the fifth Astronomer Royal, was, like Bliss, a close friend of Bradley's. He was the third son of a wealthy country gentleman, Edmund Maskelyne, of Purton, in Wiltshire. Maskelyne was born in London, October 6, 1732, and was educated at Westminster School. Thence he proceeded to Cambridge, where he graduated seventh Wrangler in 1754. He was ordained to the curacy of Barnet in 1755, and, twenty years later, was presented by his nephew, Lord Clive, to the living of Shrawardine, in Shropshire. In 1782 he was presented by his college to the Rectory of North Runcton, Norfolk.
The event which turned his thoughts in the direction of astronomy was the solar eclipse of July 25, 1748; and about the time that he was appointed to the curacy of Barnet he became acquainted with Bradley, then the Astronomer Royal, to whom he gave great assistance in the preparation of his table of refractions.
Like Halley before him, he made an astronomical expedition to the island of St. Helena. This was for the special purpose of observing the transit of Venus of June 6, 1761, Bradley having induced the Royal Society to send him out for that purpose. Here he stayed ten months, and made many observations. But though the transit of Venus was his special object, it was not the chief result of the expedition: not because clouds hindered his observations, but because the voyage gave him the especial bent of his life.
Halley had actually held a captain's commission in the Royal Navy, and commanded a ship; Maskelyne, more than any of the Astronomers Royal before or since, made the improvement of the practical business of navigation his chief aim. None of all the incumbents of the office kept its original charter—'To find the so much desired Longitude at Sea, for the perfecting the Art of Navigation,' so closely before him.
The solution of the problem was at hand at this time—its solution in two different ways. On the one hand, the offer by the Government of a reward of £20,000 for a clock or watch which should go so perfectly at sea, notwithstanding the tossing of the ship and the wide changes of temperature to which it might be exposed, that the navigator might at any moment learn the true Greenwich time from it, had brought out the invention of Harrison's time-keeper; on the other hand, the great improvement that had now taken place in the computation of tables of the moon's motion, and the more accurate star-catalogues now procurable, had made the method of 'lunars,'
[89] suggested a hundred and thirty years before by the Frenchman, Morin, and others, a practicable one.
In principle, the method of finding the longitude from 'lunars,' that is to say, from measurements of the distances between the moon and certain stars, is an exceedingly simple one. In actual practice, it involves a very toilsome calculation, beside exact and careful observation. The principle, as already mentioned, is simply this: The moon travels round the sky, making a complete circuit of the heavens in between twenty-seven and twenty-eight days. It thus moves amongst the stars, roughly speaking, its own diameter, in about an hour. When once its movements were sufficiently well known to be exactly predicted, almanacs could be drawn up in which the Greenwich time of its reaching any definite point of the sky could be predicted long beforehand; or, what comes to the same thing, its distances from a number of suitable stars could be given for definite intervals of Greenwich time. It is only necessary, then, to measure the distances between the moon and some of these stars, and by comparing them with the distances given in the almanac, the exact time at Greenwich can be inferred. As has been already pointed out, the determination of the latitude of the ship and of the local time at any place where the ship is, is not by any means so difficult a matter; but the local time being known and the Greenwich time, the difference between these gives the longitude; and the latitude having been also ascertained, the exact position of the ship is known.
There are, of course, difficulties in the way of[90] working out this method. One is, that whilst it takes the sun but twenty-four hours to move round the sky from one noon to the next, and consequently its movements, from which the local time is inferred, are fairly rapid, the moon takes nearly twenty-eight days to move amongst the stars from the neighbourhood of one particular star round to that particular star again. Consequently, it is much easier to determine the local time with a given degree of exactness than the Greenwich time; it is something like the difference of reading a clock from both hands and from the hour hand alone.
There are other difficulties in the case which make the computation a long and laborious one, and difficult in that sense; but they do not otherwise affect its practicability.
During this voyage to St. Helena, both when outward bound and when returning, Maskelyne gave the method of 'lunars' a very thorough testing, and convinced himself that it was capable of giving the information required. For by this time the improvement of the sextant, or quadrant as it then was, by the introduction of a second mirror, by Hadley, had rendered the actual observation at sea of lunar distances, and of altitudes generally, a much more exact operation.
This conclusion he put at once to practical effect, and, in 1763, he published the British Mariner's Guide, a handbook for the determination of the longitude at sea by the method of lunars.
At the same time, the other method, that by the time-keeper or chronometer, was practically tested[91] by him. The time-keeper constructed by John Harrison had been tested by a voyage to Jamaica in 1761, and now, in 1763, another time-keeper was tested in a voyage to Barbadoes. Charles Green, the assistant at Greenwich Observatory, was sent in charge of the chronometer, and Maskelyne went with him to test its performance, in the capacity of chaplain to his Majesty's ship Louisa.
The position which Maskelyne had already won for himself as a practical astronomer, and the intimate relations into which he had entered with Bradley and Bliss, made his appointment to the Astronomer Royalship, on the death of the latter, most suitable.[92] At once he bent his mind to the completion of the revolution in nautical astronomy which his British Mariner's Guide had inaugurated, and in the year after his appointment he published the first number of the Nautical Almanac, together with a volume entitled, Tables Requisite to be Used with the Nautical Ephemeris, the value of which was so instantly appreciated, that 10,000 copies were sold at once.
The Nautical Almanac was Maskelyne's greatest work, and it must be remembered that he carried it on from this time up to the day of his death—truly a formidable addition to the routine labours of an Astronomer Royal who had but a single assistant on his staff. The Nautical Almanac was, however, in the main not computed at the Observatory; the calculations were effected by computers living in different parts of the country, the work being done in duplicate, on the principle which Flamsteed had inaugurated in the preparation of his Historia Cœlestis.
Maskelyne's next service to science was almost as important. He arranged that the regular and systematic publication of the observations made at Greenwich should be a distinct part of the duties of an Astronomer Royal, and he procured an arrangement by which a special fund was set apart by the Royal Society for printing them. His observations covering the years 1776 to 1811 fill four large folio volumes, and though, as already stated, he had but one assistant, they are 90,000 in number. Thus it was Maskelyne who first rendered effective the design which Charles II. had in the establishment of the Observatory. Flamsteed and Halley had been too[93] jealous of their own observations to publish; Bradley's observations—though he himself was entirely free from this jealousy—were made, after his death, the subject of litigation by his heirs and representatives, who claimed an absolute property in them, a claim which the Government finally allowed. None of the three, however much their work ultimately tended to the improvement of the art of navigation, made that their first object. Whereas Maskelyne set this most eminently practical object in the forefront, and so gave to the Royal Observatory, which under his predecessors somewhat resembled a private observatory, its distinctive characteristics of a public institution.
It fell to Maskelyne to have to advise the Government as to the assignment of their great reward of £20,000 for the discovery of the longitude at sea. Maskelyne, while reporting favourably of the behaviour of Harrison's time-keeper, considered that the method of 'lunars' was far too important to be ignored, and he therefore recommended that half the sum should be given to Harrison for his watch, whilst the other half was awarded for the lunar tables which Mayer, before his death, had sent to the Board of Longitude. This decision, though there can be no doubt it was the right one, led to much dissatisfaction on the part of Harrison, who urged his claim for the whole grant very vigorously; and eventually the whole £20,000 was paid him. The whole question of rewards to chronometer-makers must have been one which caused Maskelyne much vexation. He was made the subject of a bitter and most[94] voluminous attack by Thomas Mudge, for having preferred the work of Arnold and Earnshaw to his own.
Otherwise his reign at the Observatory seems to have been a singularly peaceful one, and there is little to record about it beyond the patient prosecution, year by year, of an immense amount of sober, practical work. To Maskelyne, however, we owe the practice of taking a transit of a star over five wires instead of over one, and he provided the transit instrument with a sliding eye-piece, to get over the difficulty of the displacement which might ensue if the star were observed askew when out of the centre of the field. To Maskelyne, too, we owe in a pre-eminent degree the orderly form of recording, reducing, and printing the observations. Much of the work in this direction which is generally ascribed to Airy was really due to Maskelyne. Indeed, without a wonderful gift of organization, it would have been impossible to plan and to carry the Nautical Almanac.
Beside the editing of various works intended for use in nautical astronomy or in general computation, the chief events of his long reign at Greenwich were the transit of Venus in 1769, which he himself observed, and for which he issued instructions in the Nautical Almanac; and his expedition in 1774 to Scotland, where he measured the deviation of the plumb-line from the vertical caused by the attraction of the mountain Schiehallion, deducing therefrom the mean density of the earth to be four and a half times that of water.
He died at the Observatory, February 9, 1811, aged 79, leaving but one child, a daughter, who
[97] married Mr. Anthony Mervin Story, to whom she brought the family estates in Wiltshire, inherited by Maskelyne on the deaths of his elder brothers, and, in consequence, Mr. Story added the name of Maskelyne to his own.
Maskelyne's character and policy as Astronomer Royal have been sufficiently dwelt upon. His private character was mild, amiable, and generous. 'Every astronomer, every man of learning, found in him a brother;' and, in particular, when the French Revolution drove some French astronomers to this country to find a refuge, they received from the Astronomer Royal the kindest reception and most delicate assistance.
Maskelyne added no instrument to the Observatory during his reign, though he improved Bradley's transit materially. He designed the mural circle, but it was not completed until after his death. His additions to the Observatory buildings consisted of three new rooms in the Astronomer Royal's house, and the present transit circle room.
John Pond was recommended by Maskelyne as his successor at Greenwich. At the time of his succession he was forty-four years of age, having been born in 1767. He was educated at Trinity College, Cambridge, and then spent some considerable time travelling in the south of Europe and Egypt. On his return home he settled at Westbury, where he erected an altazimuth by Troughton, with a two-and-a-half-foot circle. A born observer, his observations of the declinations of some of the principal[98] fixed stars showed that the instrument which Maskelyne was using at Greenwich—the quadrant by Bird—could no longer be trusted. Maskelyne, in consequence, ordered a six-foot mural circle from Troughton, but did not live to see it installed, and in 1816 this was supplemented by Troughton's transit instrument of five inches aperture and ten feet focal length.
The introduction of these two important instruments, and of other new instruments, together with new methods of observation, form one of the chief characteristics of Pond's administration. Under this head must be specially mentioned the introduction of the mercury trough, both for determining the position of the vertical, and for obtaining a check upon the flexure of the mural circle in different positions; and the use in combination of a pair of mural circles for determining the declinations of stars.
Another characteristic of his reign was that under him there was the first attempt to give the Astronomer Royal a salary somewhat higher than that of a mechanic, and to support him with an adequate staff of assistants. His salary was fixed at £600 a year, and the single assistant of Maskelyne was increased to six.
This multiplication of assistants was for the purpose of multiplying observations, for Pond was the first astronomer to recognize the importance of greatly increasing the number of all observations upon which the fundamental data of astronomy were to be based.
In 1833 he finished his standard catalogue of 1113 stars, at that time the fullest of any catalogue prepared on the same scale of accuracy. 'It is not too much to say,' was the verdict of the Royal Astronomical Society, 'that meridian sidereal observation owes more to him than to all his countrymen put together since the time of Bradley.'
A yet higher testimony to the exactness of his work is given by his successor, Airy.
'The points upon which, in my opinion, Mr. Pond's claims to the gratitude of astronomers are founded, are principally the following. First and chief, the accuracy which he introduced into all the principal observations. This is a thing which, from its nature, it is extremely difficult to estimate now, so long after the change has been made; and I can only say that, so far as I can ascertain from books, the change is one of very great extent; for certainty and accuracy, astronomy is quite a different thing from what it was, and this is mainly due to Mr. Pond.'
The same authority eulogizes him further for his laborious working out of every conceivable cause or indication of error in his declination instruments, for the system which he introduced in the observation of transits, for the thoroughness with which he determined all his fundamental data, and for the regularity which he infused into the Greenwich observations.
One result of this great increase of accuracy was that Pond was able at once authoritatively to discard the erroneous stellar parallaxes that had been announced by Brinkley, Royal Astronomer for Ireland.
But Pond's administration was open, in several particulars, to serious censure, and the Board of[100] Visitors, which had been for many years but a committee of the Royal Society, but which had recently been reconstituted, proved its value and efficiency by the remonstrances which it addressed to him, and which eventually brought about his resignation. His personal skill and insight as an observer were of the highest order; but either from lack of interest or failing health, he absented himself almost entirely from the Observatory in later years, visiting it only every ninth or tenth day. He had caused the staff of assistants to be increased from one to six, but had stipulated that the men supplied to him should be 'drudges.' His minute on the subject ran—
'I want indefatigable, hard-working, and, above all, obedient drudges (for so I must call them, although they are drudges of a superior order), men who will be contented to pass half their day in using their hands and eyes in the mechanical act of observing, and the remainder of it in the dull process of calculation.'
This was a fatal mistake, and one which it is very hard to understand how any one with a real interest in the science could have made. Men who had the spirit of 'drudges,' to whom observation was a mere 'mechanical act,' and calculation a 'dull process,' were not likely to maintain the honour of the Observatory, particularly under an absentee Astronomer Royal. Pond tried to overcome the difficulty by devising rules for their guidance of iron rigidity. The result was that after his resignation, in 1835, the First Lord and the Secretary of the Admiralty expressed their feeling to Airy, Pond's successor, 'that the Observatory had fallen into such a state of[101] disrepute that the whole establishment should be cleared out.' A further evil was the excessive development of chronometer business, so as practically to swamp the real work of the Observatory, whilst the prices paid for the chronometers at this time were often much larger than would have been the case under a more business-like administration.
With all his merits, therefore, as an observer, the administration of Pond was, in some respects, the least satisfactory of all that the Observatory has known, and he alone of all the Astronomers Royal retired under pressure. He did not long survive his resignation, dying in September, 1836. He was buried by the side of Halley, in the churchyard at Lee.
Of Pond's instruments, the Observatory retains the fine transit instrument which was constructed by Troughton at his direction, and the mural circle, designed by Maskelyne, but which Pond was the first to use. Both of these have, of course, long been obsolete, and now hang on the walls of the transit room. The small equatorial, called, after its donor, the Shuckburgh equatorial, was also added in Pond's day, and though practically never used, still remains mounted in its special dome.
CHAPTER IV
AIRY
One hundred and sixty years from the day when Flamsteed laid the foundation stone of the Observatory, the Royal Warrant under the sign manual was issued, appointing the seventh and strongest of the Astronomers Royal, August 11, 1835. He actually entered on his office in the following October, but did not remove to the Observatory until the end of the year.
George Biddell Airy was born at Alnwick, in Northumberland, on July 27, 1801. His father was William Airy, of Luddington, in Lincolnshire, a collector of excise; his mother was the daughter of George Biddell, a well-to-do farmer, of Playford, near Ipswich. He was educated at the Grammar School, Colchester, and so distinguished himself there that although his father was at this time very straitened in his circumstances, it was resolved that young Airy should go to Cambridge. Here he was entered as sizar at Trinity College, and his robust, self-reliant character was seen in the promptness with which he rendered himself independent of all pecuniary help from his relatives. In 1823 he graduated as Bachelor
[105] of Arts, being Senior Wrangler and Smith's prizeman, entirely distancing all other men of his year. He had already begun to pay attention to astronomy, at first from the side of optics, to the study of which he had been very early attracted; a paper of his on the achromatism of eye-pieces and microscopes, written in 1824, being one of especial value. In 1826 he attempted to determine 'the diminution of gravity in a deep mine'—that of Dolcoath, in Cornwall. In the winter of 1823-24 he was invited to London by Mr. (afterwards Sir) James South, who took him, amongst other places, to Greenwich Observatory, and gave him his first introduction to practical astronomy. In 1826 he was appointed Lucasian Professor at Cambridge, and in 1828, Plumian Professor, with the charge of the new University Observatory. Prior to his election he had definitely told the electors that the salary proposed was not sufficient for him to undertake the responsibility of the Observatory. He followed this up by a formal application for an increase, which created not a little commotion at the time, the action being so unprecedented; and after a delay of a little over a year he obtained what he had asked for. The delay gave rise, however, to the remark of a local wit, that the University had given 'to Airy, nothing, a local habitation and a name.'
The seven years which he spent in the Cambridge Observatory were the best possible preparation for that greater charge which he was to assume later. When he entered on his duties the Observatory had been completed four years, but no observations had[106] been published; there was no assistant, and the only instruments were a couple of good clocks and a transit instrument. But Airy set to work at once with so much energy that the observations for 1828 were published early in the following year, and he had very quickly worked out the best methods for correcting and reducing his observations. In 1829 an assistant was granted to him, in 1833 a second, and in the latter year Mr. Baldrey, the senior assistant, observed about 5000 transits, and Mr. Glaisher, the junior, about the same number of zenith distances.
A syndicate had been appointed at Cambridge for the purpose of visiting the Observatory once in each term, and making an annual report to the senate. A smaller-minded and less acute man than Airy might have resented such an arrangement. He, on the contrary, threw himself heartily into it, and made such formal written reports to the syndicate as best helped them in the performance of their duty, and at the same time secured for the Observatory the support and assistance which from time to time it required. On his appointment to Greenwich, he at once entered into the same relations to the Board of Visitors of that Observatory, and from that time forth the friction that had occasionally existed between the Board and the Astronomer Royal in the past entirely ceased. The Board was henceforth no longer a body whose chief function was to reprove, to check, or to quicken the Astronomer Royal, but rather a company of experts, before whom he might lay the necessities of the Observatory, that they in turn might present them to the Government.
Such representations were not likely to be in vain. For, as Mr. Sheepshanks has left on record—
'When Mr. Airy wants to carry anything into effect by Government assistance, he states, clearly and briefly, why he wants it; what advantages he expects from it; and what is the probable expense. He also engages to direct and superintend the execution, making himself personally responsible, and giving his labour gratis. When he has obtained permission (which is very seldom refused), he arranges everything with extraordinary promptitude and foresight, conquers his difficulties by storm, and presents his results and his accounts in perfect order, before men like ... or myself would have made up our minds about the preliminaries. Now, men in office naturally like persons of this stamp. There is no trouble, no responsibility, no delay, no inquiries in the House; the matter is done, paid for, and published, before the seekers of a grievance can find an opportunity to be heard. This mode of proceeding is better relished by busy statesmen than recommendations from influential noblemen or fashionable ladies.'
His first action towards the Board was, however, a very bold and independent one. He made strong representations on the subject of the growth of the chronometer business, which proved displeasing to the Hydrographer, Captain Beaufort, who was one of the official visitors, and by his influence the report was not printed. Airy 'kept it, and succeeding reports, safe for three years, and then the Board of Visitors agreed to print them, and four reports were printed together, and bound with the Greenwich Observations of 1838.'
With the completion of arrangements which put the chronometer business in proper subordination to the scientific charge of the Observatory, Airy was[108] free to push forward its development on the lines which he had already marked out for himself. To go through these in detail is simply to describe the Observatory as he left it. Little by little he entirely renovated the equipment. Greatly as Pond had improved the instruments of the Observatory, Airy carried that work much further still. Though he did not observe much himself, and was not Pond's equal in the actual handling of a telescope, he had a great mechanical gift, and the detail in its minutest degree of every telescope set up during his long reign was his own design.
In the work of reduction he introduced the use of printed skeleton forms, to which Pond had been a stranger. The publication of the Greenwich results was carried on with the utmost regularity; and, in striking contrast to the reluctance of Flamsteed and Halley, he was always most prompt in communicating any observations to every applicant who could show cause for his request for them.
It is most difficult to give any adequate impression of his far-reaching ability and measureless activity. Perhaps the best idea of these qualities may be obtained from a study of his autobiography, edited and published some four years after his death by his son. The book, to any one who was not personally acquainted with Airy, is heavy and monotonous, chiefly for the reason that its 400 pages are little but a mere catalogue of the works which he undertook and carried through; and catalogues, except to the specialist, are the dullest of reading. To enter into the details of his work might fill a library.
As Astronomer Royal he seems to have inherited and summed up all the great qualities of his predecessors: Flamsteed's methodical habits and unflagging industry; Halley's interest in the lunar theory; Bradley's devotion to star observation and catalogue making; Maskelyne's promptitude in publishing, and keen interest in practical navigation; Pond's refinement of observation. Nor did he allow this inheritance to be merely metaphorical; he made it an actual reality. He discussed, reduced, and published, in forms suitable for use and comparison to-day, the whole vast mass of planetary and lunar observations made at the Royal Observatory from the year 1760 to his own accession, a work of prodigious labour, but of proportionate importance. Airy has been accused—and with some reason—of being a strong, selfish, aggressive man; yet nothing can show more clearly than this great work how thoroughly he placed the fame and usefulness of the Observatory before all personal considerations. With far less labour he could have carried on a dozen investigations that would have brought him more fame than this great enterprise, the purpose of which was to render the work of his predecessors of the highest possible use. The light in which he regarded his office may best be expressed in his own words:—
'The Observatory was expressly built for the aid of astronomy and navigation, for promoting methods of determining longitude at sea, and (as the circumstances that led to its foundation show) more especially for determination of the moon's motions. All these imply, as their first step, the formation of accurate catalogues of stars, and the determination of the fundamental elements of the[112] solar system. These objects have been steadily pursued from the foundation of the Observatory; in one way by Flamsteed; in another way by Halley, and by Bradley in the earlier part of his career; in a third form by Bradley in his later years; by Maskelyne (who contributed most powerfully both to lunar and to chronometric nautical astronomy), and for a time by Pond; then with improved instruments by Pond, and by myself for some years; and subsequently, with the instruments now in use. It has been invariably my own intention to maintain the principles of the long-established system in perfect integrity; varying the instruments, the modes of employing them, and the modes of utilizing the observations of calculation and publication, as the progress of science might seem to require.'
The result of this keen appreciation of the essential continuity of the Astronomer Royalship has been that it is to Airy, more than to any of his predecessors, or than to all of them put together, that the high reputation of Greenwich Observatory is due. Professor Newcomb, the greatest living authority on the subject outside our own land—and other great foreign astronomers have independently pronounced the same verdict—has said:—
'The most useful branch of astronomy has hitherto been that which, treating of the positions and motions of the heavenly bodies, is practically applied to the determination of geographical positions on land and at sea. The Greenwich Observatory has, during the past century, been so far the largest contributor in this direction as to give rise to the remark that, if this branch of astronomy were entirely lost, it could be reconstructed from the Greenwich observations alone.'
Early in 1836 Airy proposed to the Board of Visitors the creation of the Magnetic and Meteorological department of the Observatory, and in 1840[113] a system of regular two-hourly observations was set on foot. This was the first great enlargement of programme for the Observatory beyond the original one expressed in Flamsteed's warrant. It was followed in 1873 with the formation of the Solar Photographic department, to which the Spectroscope was added a little later.
Though he had objected strongly on his first coming to the Observatory to the excessive time devoted to the merely commercial side of the care of chronometers, yet the perfecting of these instruments was one that he had much at heart, and many recent appliances are either of his own invention or are due to suggestions which he threw out.
Much work lying outside the Observatory, and yet intimately connected with it, was carried out either by him or in accordance with his directions. The transit of Venus expeditions of 1874, the delimitation of the boundary line between Canada and the United States, and, later, that of the Oregon boundary; the determination of the longitudes of Valencia, Cambridge, Edinburgh, Brussels, and Paris; assistance in the determination of the longitude of Altona—all came under Airy's direction. Nor did he neglect expeditions in connection with what we would now call the physical side of astronomy. On three occasions, 1842, 1851, and 1860, he himself personally took part in successful eclipse expeditions. The determination of the increase of gravity observable in the descent of a deep mine was also the subject of another expedition, to the Harton Colliery, near South Shields.
But with all these, and many other inquiries—for he was the confidential adviser of the Government in a vast number of subjects: lighthouses, railways, standard weights and measures, drainage, bridges—he yet always kept the original objects of the Observatory in the very first place. It was in order to get more frequent observations of the moon that he had the altazimuth erected, which was completed in May, 1847. This was followed, in 1851, by the transit circle, as he had long felt the need for more powerful light grasp in the fundamental instrument of the Observatory. The transit circle took the place both of the old transit instrument and of the mural circle. Above all, he arranged for the observations of moon and stars to be carried out with practical continuity. The observations were made and reduced at once, and published in such a way that any one wishing to discuss them afresh could for himself go over every step of the reduction from the commencement, and could see precisely what had been done.
The greatest addition made to the equipment of the Observatory in Airy's day was the erection of the 123/4-inch Merz equatorial, which proved of great service when spectroscopy became a department of the Observatory.
So strong and gifted a man as Airy was bound to make enemies, and at different times of his life bitter attacks were made on him from one quarter or another. One of these, curiously enough, was from Sir James South, the man who, as he said, first introduced him to practical astronomy. Later came[115] the discovery of Neptune, and Airy was subjected to much bitter criticism, since, as it appeared on the surface, it was owing to his supineness that Adams[116] missed being held the sole discoverer of the new planet, and narrowly missed all credit for it altogether. Last of all was the vehement attack made upon him by Richard Anthony Proctor, in connection with his preparations for the transit of Venus. All such attacks, however, simply realized the old fable of the viper and the file. Attacks which would have agonized Flamsteed's every nerve, and have called forth full and dignified rejoinders from Maskelyne, were absolutely and entirely disregarded by Airy. He had done his duty, and in his own estimation—and, it should be added, in the estimation of those best qualified to judge—had done it well. He was perfectly satisfied with himself, and what other people thought or said about him influenced him no more than the opinions of the inhabitants of Saturn.
But great as Airy was, he had the defects of his qualities, and some of these were serious. His love of method and order was often carried to an absurd extreme, and much of the time of one of the greatest intellects of the century was often devoted to doing what a boy at fifteen shillings a week could have done as well, or better. The story has often been told, and it is exactly typical of him, that on one occasion he devoted an entire afternoon to himself labelling a number of wooden cases 'empty,' it so happening that the routine of the establishment kept every one else engaged at the time. His friend Dr. Morgan jocularly said that if Airy wiped his pen on a piece of blotting-paper he would duly endorse the blotting-paper with the date and particulars of its[117] use, and file it away amongst his papers. His mind had that consummate grasp of detail which is characteristic of great organizers, but the details acquired for him an importance almost equal to the great principles, and the statement that he had put a new pane of glass into a window would figure as prominently in his annual report to the Board of Visitors as the construction of the new transit circle. His son remarks of him that 'in his last days he seemed to be more anxious to put letters which he received into their proper place for reference than even to master their contents,' his system having grown with him from being a means to an end, to becoming the end itself.
So, too, his regulation of his subordinates was, especially in his earlier days, despotic in the extreme—despotic to an extent which would scarcely be tolerated in the present day, and which was the cause of not a little serious suffering to some of his staff, whom, at that time, he looked upon in the true spirit of Pond, as mere mechanical 'drudges.' For thirty-five years of his administration the salaries of his assistants remained discreditably low, and his treatment of the supernumerary members of his staff would now probably be characterized as 'remorseless sweating.' The unfortunate boys who carried out the computations of the great lunar reductions were kept at their desks from eight in the morning till eight at night, without the slightest intermission, except an hour at midday. As an example of the extreme detail of the oversight which he exercised over his assistants, it may be mentioned that he drew up for[118] each one of those who took part in the Harton Colliery experiment, instructions, telling them by what trains to travel, where to change, and so forth, with the same minuteness that one might for a child who was taking his first journey alone; and he himself packed up soap and towels with the instruments, lest his astronomers should find themselves, in Co. Durham, out of reach of these necessaries of civilization.
A regime so essentially personal may indeed have been necessary after Pond's administration, and to give the Observatory a fresh start. But it would not have been to the advantage of the Observatory, had it become a permanent feature of its administration, as it militated—was almost avowedly intended to militate—against the growth of real zeal and intelligence in the staff, and necessarily occasioned labour and discomfort out of proportion to the results obtained. Fortunately, in Airy's later years, the extension of the work of the Observatory, a slight failing in his own powers, and the efforts he was devoting to the working out of the lunar theory, compelled him to relax something of that microscopic imperiousness which had been the chief characteristic of his rule for so long.
Airy had, in the fullest degree, the true spirit of the public servant; his sense of duty to the State was very high. He was always ready to undertake any duty which he felt to be of public usefulness, and many of these he discharged without fee or reward.
So great an astronomer was necessarily most highly esteemed by astronomers. He was President[119] of the Royal Society for two years; he was five times President of the Royal Astronomical Society, and twice received its gold medal, beside a special testimonial for his reduction of the Greenwich lunar observations. From the Royal Society he received the Copley medal and the Royal medal, beside honorary titles from the Universities of Oxford, Cambridge, and Edinburgh. So invaluable a public servant, he received the distinction of a Knight Commandership of the Bath in 1872. He had been repeatedly offered knighthood before, but had not thought it well to receive it. He was in the receipt of decorations also from a great number of foreign countries; for, for many years, he was looked up to, not only by English astronomers, but by scientific men in all countries, as the very head and representative of his science.
And he also received a more popular appreciation—and most justly so. For whilst no one could have less of the arts of the ordinary popularizer about him, no one has ever given popular lectures on astronomy which more fully corresponded to the ideal of what such should be than Airy's six lectures to working men, delivered at Ipswich. And we may count the bestowal upon him of the honorary freedom of the City of London, in 1875, as one of the tokens that his services in this direction had not been unappreciated.
During the last seven years of his official career he undertook the working out of a lunar theory, and, to allow himself more leisure for its completion, he resigned his position August 15, 1881, after forty-six[120] years of office. He was now eighty years of age, and he took up his residence at the White House, just outside Greenwich Park. He resided there till his death, more than ten years later—January 2, 1892.
Airy was succeeded in the Astronomer Royalship by the present and eighth holder of the office, W. H. M. Christie. He was born at Woolwich, in 1845, his father having been Professor Samuel Hunter Christie, F.R.S. He was educated at King's College, London, and Trinity College, Cambridge, graduating as fourth Wrangler in 1868. In 1870 he was appointed chief assistant at Greenwich, in succession to Mr. Stone, who had become her Majesty's astronomer at the Cape, and in 1881 he succeeded Airy as Astronomer Royal.
During Mr. Christie's office, the two new departments of the Astrographic Chart and Double-star observations have come into being. The following buildings have been erected under his administration: the great New Observatory in the south ground, the New Altazimuth, the New Library, nearly opposite to it, the Transit Pavilion, the porter's lodge, and the Magnetic Pavilion out in the Park. Whilst in the old buildings the Astrographic dome has been added, and the Upper and Lower Computing rooms have been rebuilt and enlarged. As to the instruments, the 28-inch refractor, the astrographic twin telescope, the new altazimuth, the 26-inch and 9-inch Thompson photographic refractors, and the 30-inch reflector are all additions during the present reign. Roughly speaking, therefore,
[123] we may say that three-fourths of the present Observatory has been added during the nineteen years of the present Astronomer Royal. One exceedingly important improvement should not be overlooked. Airy observed little himself whilst at Greenwich, and had an inadequate idea of the necessity for room in a dome and breadth in a shutter-opening. With the sole exception, perhaps, of the transit circle, every instrument set up by Airy was crammed into too small a dome or looked out through too narrow an opening. The increase of shutter-opening of the newer domes may be well seen by contrasting, say, the old altazimuth or the Sheepshanks dome with that of the astrographic. This reform has had much to do with the success of later work.
CHAPTER V
THE OBSERVATORY BUILDINGS
Like a living organism, Greenwich Observatory bears the record of its life-history in its structure. It was not one of those favoured institutions that have sprung complete and fully equipped from the liberality of some great king or private millionaire. As we have seen, it was originally established on the most modest—not to say meagre—scale, and has been enlarged just as it has been absolutely necessary. To quote again from Professor Newcomb—
'Whenever any part of it was found insufficient for its purpose, new rooms were built for the special object in view, and thus it has been growing from the beginning by a process as natural and simple as that of the growth of a tree. Even now the very value of its structure is less than that of several other public observatories, though it eclipses them all in the results of its work.'
Entering the courtyard—an enclosure some eighty feet deep by ninety feet in extreme breadth—by the great gate, we see before us Flamsteed House, the original building of the Observatory. Flamsteed's little domain was only some twenty-seven yards wide by fifty deep, and for buildings comprised little beyond a small dwelling-house on the ground floor, and one fine room above it. This room—the original[125] Greenwich Observatory—still remains, and is used as a council room by the official Board of Visitors, who come down to the Observatory on the first Saturday in June, to examine into its condition and to receive the Astronomer Royal's report. The room is called, from its shape, the Octagon Room, and is well known to Londoners from the great north window which looks out straight over the river between the twin domes of the Hospital.
In Bradley's time, about 1749, the first extension of the domains of the Observatory took place to the south and east of the original building, the direction in which, on the whole, all subsequent extensions have taken place, owing to the fact that the original building was constructed at the extremity of what Sir George Airy was accustomed to call a 'peninsula'—a projecting spur of the Blackheath plateau, from which the ground falls away very sharply on three sides and on part of the fourth.
The Observatory domain at present is fully two hundred yards in greatest length, with an average breadth of about sixty. Nearly the whole of this accession took place under the directorates of Pond and Airy. The present instruments are, therefore, as a rule, the more modern in direct proportion to their distance from the Octagon Room—the old original Observatory. There is one notable exception. The very first extension of the Observatory buildings, made in the time of Halley, the second Astronomer Royal, consisted in the setting up of a strong pier, to carry two quadrant telescopes. The pier still remains, but now forms the base of the support of[126] the twin telescopes devoted to the photographic survey of the heavens for the International Chart.
Standing just within the gate of the courtyard, and looking westward, that is toward Flamsteed House, we have immediately on our right hand the porter's lodge; a little farther forward, also on the right, the Transit Pavilion, a small building sheltering a portable transit instrument; and farther forward, still on the right, the entrance to the Chronograph Room. Above the Chronograph Room is a little, inconveniently-placed dome, containing a small equatorially-mounted telescope, known as the Shuckburgh. Beyond the Chronograph Room a door opens on to the North Terrace, over which is seen the great north window of the Octagon Room. Close by the door of the Chronograph Room a great wooden staircase rises to the roof of the main building. It is not an attractive-looking ascent, as the steps overlap inconveniently. Still, there is no record of an accident upon them, and those who venture on the climb to the roof, where are placed the anemometers and the turret carrying the time-ball, which is dropped daily at 1 p.m., will be well repaid by the splendid view of the river which is there afforded to them.
Passing under this staircase, on the wall by its side is seen the following inscription:—
Carolus IIs Rex Optimus
Astronomiæ et Nauticæ artis
Patronus Maximus
Speculam hanc in utriusque commodum
fecit
Anno DNI MDCLXXVI. Regni sui XXVIII.
Curante Iona Moore milite
R. T. S. G.
In the extreme angle of the courtyard is the entrance to the mean solar clock cupboard, and to the staircase leading up to the Octagon Room. At the head of this staircase in a small closet is the winch for winding up the time-ball.
Coming back into the courtyard, and crossing the face of the Astronomer Royal's private house, the range of buildings is reached which form the left hand or south side of the enclosure. Entering the first of these, we find ourselves in the Lower Computing Room, which is devoted to the 'Time Department.' The next room which opens out of it, as we turn eastwards, was Bradley's Transit Room, but is now used for the storage of chronometers. Passing through Bradley's Transit Room, we come to the present Transit Room, which brings us close to the great gate. The range of buildings is, however, continued somewhat farther, containing on the ground floor some small sitting-rooms and a fire-proof room for records.
Turning back to the Lower Computing Room, we notice in it the stone pier, already alluded to, which was set up by Halley, and formed the first addition to the original Observatory of Flamsteed. The Lower Computing Room itself and Bradley's Transit Room were due to the Astronomer after which the latter is named. An iron spiral staircase in the middle of the Lower Computing Room leads up to the Upper Computing Room, and above that to the Astrographic dome, so called because the twin telescope housed therein is devoted to the work of the Astrographic Chart—a chart of the entire sky to be made by eighteen co-operating observatories by means of photography. In this way it is intended to secure a record of the places of far more stars than could be done by the ordinary methods, and in this project Greenwich has necessarily taken a premier
[131] place. This is a work which, whilst it is the legitimate and natural outcome of the original purpose of the Observatory, is yet pushed beyond what is necessary for any mere utilitarian assistance to navigation. For the sailor it will always be sufficient to know the places of a mere handful of the brightest stars, and the vast majority of those in the great photographic map will never be visible in the little portable telescope of the sailor's sextant. But it will be freely admitted that in the case of an enterprise of this nature, in which the observatories of so many different nations were uniting, and which was so precisely on the lines of its original charter, though an extension of it, it was impossible for Greenwich to hold back on the plea that the work was not entirely utilitarian.
Descending again to the Lower Computing Room, and passing through it, not to the east, into Bradley's Transit Room, but through a little lobby to the south, we come upon an inconvenient wooden staircase winding round a great stone pillar with three rays. This pillar is the support of Airy's altazimuth, and very nearly marks the place where Flamsteed set up his original sextant.
Returning again to the Lower Computing Room, and passing out to the east, just in front of the Time Superintendent's desk, we enter a small passage running along the back of Bradley's Transit Room, and from this passage enter the present Transit Room near its south end. Just before reaching the Transit Room, however, we pass the Reflex Zenith Tube, a telescope of a very special kind.
Immediately outside the Transit Room is a staircase leading on the first floor to two rooms long used as libraries, and to the leads above them, on which is a small dome containing the Sheepshanks equatorial. These libraries are over the small sitting-rooms already referred to. The fire-proof Record Rooms, two stories in height, terminate this range of buildings.
Beyond the Record Rooms the boundary turns sharply south, where stands a large octagonal building surmounted by a dome of oriental appearance, a 'circular versatile roof,' as the Visitors would have called it a hundred years ago. This dome—which has been likened, according to the school of æsthetics in which its critics have been severally trained, to the Taj at Agra, a collapsed balloon, or a mammoth Spanish onion—houses the largest refractor in England, the 'South-east Equatorial' of twenty-eight inches aperture. But, though the largest that England possesses, it would appear but as a pigmy beside some of the great telescopes for which America is famous.
Beyond this dome the hollow devoted to the Astronomer Royal's private garden reduces the Observatory ground to a mere 'wasp's waist,' a narrow, inconvenient passage from the old and north observatory to the younger southern one.
The first building, as the grounds begin to widen out to the south, contains the New Altazimuth, a transit instrument which can be turned into any meridian. A library of white brick and a low wooden cruciform building—the Magnetic Observatory—follow it closely.
This latter building houses the Magnetic Department, a department which, though it lies aside from the original purposes of the Observatory, as defined in the warrant given to Flamsteed, is yet intimately connected with navigation, and was founded by Airy very early in his period of office. This deals with the observation of the changes in the force and direction of the earth's magnetism, an inquiry which the greater delicacy of modern compasses, and, in more recent times, the use of iron instead of wood in the construction of ships, has rendered imperative.
Closely associated with the Magnetic Department is the Meteorological. Weather forecasts, so necessary for the safety of shipping round our coasts, are not issued from Greenwich Observatory, any more than the Nautical Almanac is now issued from it. But just as the Observatory furnishes the astronomical data upon which the almanac is based, so also a considerable department is set apart for furnishing observations to be used by the Meteorological Office at Westminster for their daily predictions.
So far, the development of the Observatory had been along the central line of assistance to navigation. But the 'Magnetic Department' led on to a new one, which had but a secondary connection with it. It had been discovered that the extent of the daily range of the magnetic needle, and the amount of the disturbances to which it was subjected, were in close connection with the numbers and size of the spots on the sun's surface. This led to the institution of a daily photographic record of the state of the sun's
[135] surface, a record of which Greenwich has now the complete monopoly.
Key to the Plan of the Observatory on Page 134.
1. Chronograph Room.
2. Old Altazimuth Dome.
3. Safe Room.
4. Computing Room.
5. Bradley's Transit Room.
6. Transit Circle Room.
7. Assistants' Room.
8. Chief Assistant's Room.
9. Computers' Room.
10. Record Rooms.
11. Chronometer Rooms and South-east Dome.
12. Greenhouse and Outbuildings.
14. New Library.
15. Magnetic Observatory.
16. Offices.
19. Sheds.
23. Winch Room for Time-ball.
24. Porter's Lodge.
25. New Transit Pavilion.
26. New Altazimuth Pavilion.
27. Museum: New Building.
28. South Wing "
29. North Wing "
30. West Wing "
31. East Wing "
F. Rooms built for Flamsteed.
H. Added by Halley.
B. " Bradley.
M. " Maskelyne.
A. " Airy.
F'F'. Flamsteed's boundaries.
M'M'. Maskelyne's " 1790.
P'P'. Pond's " 1814.
A'A'. Airy's " 1837.
A"A". Airy's " 1868.
Beyond the Magnetic Observatory the ground widens out into an area about equal to that of the northern part, and the new building just completed, and which is now emphatically 'The Observatory,' stands clear before us. The transfer to this stately building of the computing rooms, libraries, and store rooms has been aptly described as a shift in the latitude of Greenwich Observatory, which still preserves its longitude. It may be noted that the only two buildings of any architectural pretensions in the whole range are—Flamsteed's original observatory, built by Sir Christopher Wren, and containing little beyond the octagon room, in the extreme north; and this newest building in the extreme south.
This 'New Observatory,' like the old, and like the great South-eastern tower, is an octagon in its central portion. But whilst the two other great buildings are simply octagonal, here the octagon serves only as the centre from which radiate four great wings to the four points of the compass. The building is by far the largest on the ground, but in little accord with the popular idea of an astronomer as perpetually looking through a telescope, carries but a single dome; its best rooms being set apart as 'computing rooms,' for the use of those members of the staff who are employed in the calculations and other clerical work, which form, after all, much the greater portion of the Observatory routine.
An observer with the transit instrument, for instance, will take only three or four minutes to make a complete determination of the place of a single star. But that observation will furnish work to the computers for many hours afterwards. Or, to take a photograph of the sun will occupy about five minutes in setting the instrument, whilst the actual exposure will take but the one-thousandth part of a second. But the plate, once exposed, will have to be developed, fixed, and washed; then measured, and the measures reduced, and, on the average, will provide one person with work for four days before the final results have been printed and published.
It is easy to see, then, that observing, though the first duty of the Observatory, makes the smallest demand on its time. The visitor who comes to the Observatory by day (and none are permitted to do so by night) finds the official rooms not unlike those[137] of Somerset House or Whitehall, and its occupants for the most part similarly engaged in what is, apparently, merely clerical work. An examination of the big folios would of course show that instead of being ledgers of sales of stamps, or income-tax schedules, they referred to stars, planets, and sun-spots; but for one person actively engaged at a telescope, the visitor would see a dozen writing or computing at a desk.
The staff, like the building, is the result of a gradual development, and bears traces of its life history in its composition. First comes the Astronomer Royal, the representative and successor of the original 'King's Astronomer,' the Rev. John Flamsteed. But the 'single surly and clumsy labourer,' which was all that the 'Merry Monarch' could grant for his assistance, is now represented by a large and complex body of workers; each varied class and rank of which is a relic of some stage in the progress of the Observatory to its present condition.
The following extract from the Annual Report of the Astronomer Royal to the Board of Visitors, June, 1900, describes the present personnel of the establishment:—
'The staff at the present time is thus constituted, the names in each class being arranged in alphabetical order:—
'Chief assistants—Mr. Cowell, Mr. Dyson.
'Assistants—Mr. Hollis, Mr. Lewis, Mr. Maunder, Mr. Nash, Mr. Thackeray.
'Second-class assistants—Mr. Bryant, Mr. Crommelin.
'Clerical assistant—Mr. Outhwaite.
'Established computers—Mr. Bowyer, Mr. Davidson, Mr. Edney, Mr. Furner, Mr. Rendell, and one vacancy.
'The two second-class assistants will be replaced by higher grade established computers as vacancies occur.
'Mr. Dyson and Mr. Cowell have the general superintendence of all the work of the Observatory. Mr. Maunder is charged with the heliographic photography and reductions, and with the preparation of the Library Catalogue. Mr. Lewis has charge of the time-signals and chronometers, and of the 28-inch equatorial. Mr. Thackeray superintends the miscellaneous astronomical computations, including the preparation of the new Ten-Year Catalogue. Mr. Hollis has charge of the photographic mapping of the heavens, the measurement of the plates, and the computations for the Astrographic Catalogue. Mr. Crommelin undertakes the altazimuth and Sheepshanks equatorial reductions, and Mr. Bryant the transit and meridian zenith distance reductions and time-determinations. In the magnetic and meteorological branch, Mr. Nash has charge of the whole of the work. Mr. Outhwaite acts as responsible accountant officer; has charge of the library, records, manuscripts, and stores, and conducts the official correspondence. As regards the established computers, Mr. Bowyer, Mr. Furner, Mr. Davidson, and Mr. Rendell assist Mr. Lewis, Mr. Thackeray, Mr. Hollis, and Mr. Bryant respectively, and Mr. Edney assists Mr. Nash.
'There are at the present time twenty-four supernumerary computers employed at the Observatory, ten being attached to the astronomical branch, two the chronometer branch, six to the astrographic, one to the heliographic, four to the magnetic and meteorological, and one to the clerical.
'A foreman of works, with two carpenters, and two labourers; a skilled mechanic with an assistant; a gate porter, two messengers, a watchman, a gardener, and a charwoman, are also attached to the Observatory.
'The whole number of persons regularly employed at the Observatory is fifty-three.'
The day work, as said before, is by far the greatest in amount, the 'office hours' being from[139] nine till half-past four, with an hour's interval. The arrangements for the night watches present some complications.
For many years the instruments in regular use were two only, the transit circle and the altazimuth. The arrangements for observing were simple. Four assistants divided the work between them thus: an assistant was on duty with the transit circle one day, his watch beginning about six a.m. or a little later, and ending about three the following morning; a watch of twenty-one hours in maximum length. The second day his duties were entirely computational, and were only two or three hours in length. The third day he had a full day's work on the calculations, followed by a night duty with the altazimuth. The latter instrument might give him a very easy watch or a terribly severe one. If the moon were a young one it was easy, especially if the night was clear, as in that case an hour was enough to secure the observations required.
Very different was the case with a full moon, especially in the long, often cloudy, nights of winter. Then a vigilant watch had to be kept from sunset to sunrise, so that in case of a short break in the clouds the moon might yet be observed. Such a watch was the severest (with one exception) that an assistant had to undergo.
His fourth day would then resemble his second, and with the fifth day a second cycle of his quartan fever would commence, the symptoms following each other in the same sequence as before.
Such a routine carried on with iron inflexibility[140] was exceedingly trying, as it was absolutely impossible for an observer to keep any regularity in his hours of rest or times for meals.
This routine has been considerably modified by the present Astronomer Royal, partly because the instruments now in regular daily use are five instead of two, and partly because a less stringent system has proved not merely far less wearing to the observers, but also much more prolific of results. It was impossible for a man to be at his best for long under the old régime, and from forty-six to forty-seven has been an ordinary age for an assistant to break down under the strain.
One point in which the observing work has been lightened has been in the discontinuance of the altazimuth observations at the full of the moon, another in the shortening of the hours of the transit circle watch; and a further and most important one in the arrangement that the observers with the larger instruments should have help at their work. The net result of these changes has been a most striking increase in the amount of work achieved. Thus, whilst in the year ending May 20, 1875, 3780 transits were taken with the transit circle, and 3636 determinations of north polar distance; in that ending May 10, 1895, the numbers had risen to 11,240 and 11,006 respectively, the telescope remaining precisely the same.
One principle of Airy's rule still remains. So far as possible no observer is on duty for two consecutive days, but a long day of desk work and observing is followed by a short day of desk work without observing.
It will be readily understood that with five principal telescopes in constant work and one or two minor ones, some demanding two observers, others only one, each telescope having its special programme and its special hours of work, whilst by no means every member of the staff is authorized to observe with all instruments indifferently, it becomes a somewhat intricate matter to arrange the weekly rota in strict accordance with the foregoing principle, and with the further one, that whilst a considerable amount of Sunday observing is inevitable, the average duty of an observer should be three days a week, not seven days a fortnight. There is a story, received with much reserve at Cambridge, that there was once a man at that university who had mastered all the colours and combinations of shades and colours of the various colleges and clubs. If so gifted a being ever existed, he may be paralleled by the Greenwich assistant who can predict for any future epoch the sequence of duties throughout the entire establishment. At any rate, one of the first items in the week's programme is the preparation of the rota for the week, or rather, to use an ecclesiastical term, for the 'octave,' i.e. from the Monday to the Monday following.
The special work to be carried out on any telescope is likewise a matter of programme. For the transit circle a list of the most important objects to be observed is supplied for the observer's use, and the general lines upon which the other stars are to be selected from a huge 'Working Catalogue' are well understood. With some of the other telescopes[142] the principles upon which the objects are to be selected are laid down, but the actual choice is left to the discretion of the observer at the time. There is no time for the watcher to spend in what the outsider would regard as 'discovery'; such as sweeping for comets or asteroids, hunting for variable stars, sketching planets, and so forth. Indeed, there is a story current in the Observatory that some fifty years ago, when the tide of asteroid discovery first set in, Airy found an assistant, since famous, working with a telescope on his 'off-duty' night. That stern disciplinarian asked what business the assistant had to be there on his free night, and on being told he was 'searching for new planets,' he was severely reprimanded and ordered to discontinue at once. A similar energy would not meet so gruff a discouragement to-day; but the routine work so fully occupies both staff and telescopes that an assistant may be most thoroughly devoted to his science, and yet pass a decade at the Observatory without ever seeing those 'show places' of the sky which an amateur would have run over in the first week after receiving his telescope. For example, there is no refractor in the British Isles so competent to bring out the vivid green light of the great Orion nebula—that marvellous mass of glowing, curdling, emerald cloud—or the indescribable magnificence of the myriad suns that cluster like swarming bees or the grapes of Eshcol in the constellation of Hercules; yet probably most of the staff have never seen either spectacle through it. The professional astronomer who is worth his salt will find[143] abundance of charm and interest in his work, but he will not,
consider the charm to lie mainly in the occasional sight of wonderful beauty which his work may bring to him, nor the interest in some chance phenomenon which may make his name known.
It is not every field of astronomy that is cultivated at Greenwich. The search for comets and for 'pocket planets' forms no part of its programme; and the occupation so fascinating to those who take it up, of drawing the details on the surfaces of the moon, Mars, Jupiter, or Saturn, has been but little followed. Such work is here incidental, not fundamental, and the same may be said of certain spectroscopic observations of new or variable stars, and of many similar subjects. Work such as this is most interesting to the general public, and is followed with much devotion by many amateur astronomers. For that very reason it does not form an integral part of the programme of our State observatory. But work which is necessary for the general good, or for the advancement of the science, and which demands observations carried on continuously for many years, and strict unity of instruments and methods, cannot possibly be left to chance individual zeal, and is therefore rightly made the first object at Greenwich.
Those striking discoveries which from time to time appeal strongly to the popular imagination,[144] and which have rendered so justly famous some of the great observatories of the sister continent, have not often been made here.
Its work has, none the less, been not only useful but essential. A century ago, when we were engaged in the hand-to-hand struggle with Napoleon, by far the most brilliant part of that naval war which we waged against the French, and the most productive of prize-money, was carried on by our cruisers, who captured valuable prizes in every sea. But a much greater service, indeed an absolutely vital one, was rendered to the State by those line-of-battle ships which were told off to watch the harbours wherein the French fleet was taking refuge. This was a work void of the excitement, interest, and profit of cruising. It was monotonous, wearing, and almost inglorious, but absolutely necessary to the very existence of England. So the continuance for more than two centuries of daily observations of places of moon, stars, and planets is likewise 'monotonous, wearing, and almost inglorious;' the one compensation is that it is essential to the life of astronomy.
The eight Astronomers Royal have, as already said, kept the Observatory strictly on the lines originally laid down for it, subject, of course, to that enlargement which the growth of the science has inevitably brought. But had they been inclined to change its course, the Board of Visitors has been specially appointed to bring them back to the right way. As already mentioned in the account of Flamsteed, the Board dates from 1710, when it practically consisted of the President and Council[145] of the Royal Society. Its Royal warrant lapsed on the death of Queen Anne, and was not renewed at the accession of the two following sovereigns; but in the reign of George III. a new warrant was issued under date February 22, 1765; and this was renewed at the accession of George IV. When William IV. came to the throne, the constitution of the Board was extended, so as to give a representation to the new Royal Astronomical Society, founded in 1820. The President of the Royal Society is still chairman of the Board, but the Admiralty, of which the Observatory is a department, the two Universities of Oxford and Cambridge, and the Royal Astronomical Society are all represented on it by ex officio members, and twelve other members are contributed by the Royal and Royal Astronomical Societies respectively, six by each. The first Saturday in June is the appointed day for the annual inspection by the Board, and for the presentation to it of the Astronomer Royal's Report. To this all-important business meeting has been added something of a social function, by the invitation of many well-known astronomers and the leading men of the allied sciences to inspect the results of the year, and to partake of the chocolate and cracknels, which have been the traditional refreshments offered on these occasions for a period 'whereof the memory of man runneth not to the contrary.'
CHAPTER VI
THE TIME DEPARTMENT
One day two Scotchmen stood just outside the main entrance of Greenwich Observatory, looking intently at the great twenty-four-hour clock, which is such an object of attention to the passers through the Park. 'Jock,' said one of them to the other, 'd'ye ken whaur ye are?' Jock admitted his ignorance. 'Ye are at the vara ceentre of the airth.'
Geographers tell us that there is a sense in which this statement as it stands may be accepted as true. For if the surface of the globe be divided into two hemispheres, so related to each other that the one contains as much land as possible, and the other as little, then London will occupy the centre or thereabouts of the hemisphere with most land.
This was not, however, what the Scotchman meant. He meant to tell his companion that he was standing on the prime meridian of the world, the imaginary base line from which all distances, east or west, are reckoned; in short, that he was on 'Longitude Nought.'
He was not absolutely correct, however, for the great twenty-four-hour clock does not mark the exact[147] meridian of Greenwich. To find the instrument which marks it out and defines it we must step inside the Observatory precincts, and just within the gate we see before us on the left hand a door which leads through a little lobby straight into the most important room of the whole Observatory—the Transit Room.
This room is not well adapted for representation by artist or photographer. Four broad stone pillars[148] occupy the greater part of the space, and leave little more than mere passage room beside. Two of these pillars are tall, as well as broad and massive, and stand east and west of the centre of the room, carrying between them the fundamental instrument of the Observatory, the transit circle. The optical axis of this telescope marks 'Longitude Nought,' which is further continued by a pair of telescopes, one to the north of it, the other to the south, mounted on the third and fourth of the pillars alluded to above.
This room has not always marked the meridian of Greenwich, for it stands outside the original boundary of the Observatory. But it is only a few feet to the east of the first transit instrument which was set up by Halley, the second Astronomer Royal, in the extreme N.-W. corner of the Observatory domain, a distance equivalent to very much less than one-tenth of a second of time, an utterly insensible quantity with the instruments of two hundred years ago.
It would be a long story to tell in detail how the Greenwich transit room has come to define one of the two fundamental lines that encircle the earth. The other, the equator, is fixed for us by the earth itself, and is independent of any political considerations, or of any effort or enterprise of man. But of all the infinite number of great circles which could be drawn at right angles to the equator, and passing through the north and south poles, it was not easy to select one with such an overwhelming amount of argument in its favour as to obtain a practically[149] universal acceptance. The meridians of Jerusalem and of Rome have both been urged, upon what we may call religious or sentimental grounds; that of the Great Pyramid at Ghizeh has been pressed in accordance with the fantastic delusion that the Pyramid was erected under Divine inspiration and direction; that of Ferro, in the Canaries, as being an oceanic station, well to the west of the Old World, and as giving a base line without preference or distinction for one nation rather than another.
The actual decision has been made upon no such grounds as these. It has been one of pure practical convenience, and has resulted from the amazing growth of Great Britain as a naval and commercial power. Like Tyre of old, she is 'situate at the entry of the sea, a merchant of the people for many isles,' and 'her merchants are the great men of the earth.' To tell in full, therefore, the steps by which the Greenwich meridian has overcome all others is practically to tell again, from a different standpoint, the story of the 'expansion of England.' The need for a supreme navy, the development of our empire beyond the seven seas, the vast increase of our carrying trade—these have made it necessary that Englishmen should be well supplied with maps and charts. The hydrographic and geographic surveys carried on, either officially by this country, or by Englishmen in their own private capacity, have been so numerous, complete, and far-reaching as not only to outweigh those of all other countries put together, but to induce the surveyors and explorers of not a few other countries to adopt in their work the same[150] prime meridian as that which they found in the British charts of regions bordering on those which they were themselves studying. Naturally, the meridian of Greenwich has not only been adopted for Great Britain, but also for the British possessions over-sea, and, from these, for a large number of foreign countries; whilst our American cousins retain it, an historic relic of their former political connection with us. The victories of Clive at Arcot and Plassy, of Nelson at the Nile and Trafalgar, the voyages and surveys of Cook and Flinders, and many more; the explorations of Bruce, Park, Livingstone, Speke, Cameron, and Stanley; these are some of the agencies which have tended to fix 'Longitude Nought' in the Greenwich Transit Room.
There are two somewhat different senses in which the meridian of Greenwich is the standard meridian for nearly the entire world. The first is the sense about which we have already been speaking; it constitutes the fundamental line whence distances east and west are measured, just as distances north and south are measured from the equator. But there is another, though related sense, in which it has become the standard. It gives the time to the world.
There are few questions more frequently put than, 'What time is it?' 'Can you tell me the true time?' A stickler for exactitude might reply, 'What kind of time do you mean?' 'Do you mean solar or sidereal time?' 'Apparent time or mean time?' 'Local time or standard time?' There are all these six kinds of time, but it is only within the last two generations, within, indeed, the reign of our Sovereign,[151] Queen Victoria, that the subject of the differences of most of these kinds of time has become of pressing importance to any but theorists.
In one of the public gardens of Paris a little cannon is set up with a burning-glass attached to it in such a manner that the sun itself fires the cannon as it reaches the meridian. This, of course, is the time of Paris noon—apparent noon—but it would be exceedingly imprudent of any traveller through Paris who wished, say, to catch the one o'clock express, to set his watch by the gun. For if it happened to be in February, he would find when he reached the railway station that the station clock was faster than the sun by nearly a full quarter of an hour, and that his train had gone; whilst towards the end of October or the beginning of November, he would find himself as much too soon.
Until machines for accurately measuring time were invented, apparent time—time, that is to say, given by the sun itself, as by a sun-dial—was the only time about which men knew or cared. But when reasonably good clocks and watches were made, it was very soon seen that at different times in the year there was a marked difference between sun-dial time and that shown by the clock, the reason being simply that the apparent rate of motion of the sun across the sky was not always quite the same, whilst the movement of the clock was, of course, as regular as it could be made.
This difference between time as shown by the actual sun and by a perfect clock is known as the 'equation of time.' It is least about April 15, June 15,[152] August 31, and December 25. It is greatest, the sun being after the clock, about February 11; and the sun being before the clock, about November 2. Flamsteed, before he became Astronomer Royal, investigated the question, and so clearly demonstrated the existence, cause, and amount of the equation of time as entirely to put an end to controversy on the subject.
We had thus, early in the century, the two kinds of time in common use, apparent time and mean time, or clock time. But as the sun can only be on one particular meridian at any given instant, the time as shown by the clocks in one particular town will differ from that of another town several miles to the east or west of it. It is thus noon at Moscow 1 hr. 36 min. before it is noon at Berlin, and noon at Berlin 54 min. before it is noon in London.
This was all well enough known, but occasioned no inconvenience until the introduction of railway travelling; then a curious difficulty arose. Suppose an express train was running at the rate of sixty miles an hour from London to Bristol. The guard of the train sets his watch to London time before he leaves Paddington, but if the various towns through which the train passes, Reading, Swindon, etc., each keep their own local time, he will find his watch apparently fast at each place he reaches; but on his return journey, if he sets to Bristol time before starting, he will in a similar way find it apparently slow by the Swindon, Reading, and Paddington clocks as he reaches them in succession.
It became at once necessary to settle upon one uniform system of time for use in the railway guides.[153] Apart from this, a passenger taking train, say, at Swindon, might have been very troubled to know whether the advertised time of his train was that of Exeter, the place whence it started, or Swindon, the station where he was getting in, or London, its destination. 'Railway time,' therefore, was very early fixed for the whole of Great Britain to be the same as London time, which is, of course, time as determined at Greenwich Observatory. At first it was the custom to keep at the various stations two clocks, one showing local time, the other 'railway,' or Greenwich time, or else the clocks would be provided with a double minute hand, one branch of which pointed to the time of the place, the other to the time of Greenwich.
It was soon found, however, that there was no sufficient reason for keeping up local time. Even in the extreme West of England the difference between the two only amounted to twenty-three minutes, and it was found that no practical inconvenience resulted from saying that the sun rose at twenty-three minutes past six on March 22, rather than at six o'clock. The hours of work and business were practically put twenty-three minutes earlier in the day, a change of which very few people took any notice.
Other countries besides England felt the same difficulty, and solved it in the same way, each country as a rule taking as its standard time the time of its own chief city.
There were two countries for which this expedient was not sufficient—the United States and Canada. The question was of no importance until the iron[154] road had linked the Atlantic to the Pacific in both countries. Then it became pressing. No fewer than seventy different standards prevailed in the United States only some twenty years ago. The case was a very different one here from that of England, where east and west differed in local time by only a little over twenty minutes. In North America, in the extreme case, the difference amounted to four hours, and it seemed asking too much of men to call eight o'clock in their morning, or it might be four o'clock in their afternoon, their noonday.
The device was therefore adopted of keeping the minutes and seconds the same for all places right across the continent, but of changing the hour at every 15° of longitude. The question then arose what longitude should be adopted as the standard. The Americans might very naturally have taken their standard time from their great national observatory at Washington, or from that of their chief city, New York, or of their principal central city, Chicago. But, guided partly no doubt by a desire to have their standard times correspond directly to the longitudes of their maps, and partly from a desire to fall in, if possible, with some universal time scheme, if such could be brought forward, they fixed upon the meridian of Greenwich as their ultimate reference line, and defined their various hour standards as being exactly so many hours slow of Greenwich mean time.
The decision of the United States and of Canada brought with it later a similar decision on the part of all the principal States of Europe; and Greenwich is[155] not only 'Longitude Nought' for the bulk of the civilized world, but Greenwich mean time, increased or decreased by an exact number of hours or half-hours, is the standard time all over the planet.
No; the statement requires correction. Two countries hold out, both close to our own doors. France, instead of adopting Greenwich time as such, adopts Paris time less 9 m. 21 s. (that being the precise difference in longitude between the two national observatories). Ireland disdains even such a veiled surrender, and Dublin time is the only one recognized from the Hill of Howth to far Valentia. So the distressful country preserves her old grievance, that she does not even get her time until after England has been served.
The alteration in national habits following on the adoption of this European system has had a very perceptible effect in some cases. Thus, Switzerland has adopted Mid-European time, one hour fast of Greenwich; the true local time for Berne being just half an hour later. The result of putting the working hours this thirty minutes earlier in the day has had such a noticeable effect on the consumption of gas, as to lead the gas company to contemplate agitating for a return to the old system.
Thus, Greenwich time, as well as the Greenwich meridian, has practically been adopted the world over.
It follows, then, that the determination of time is the most important duty of the Royal Observatory; and the Time Department, the one to which is entrusted the duty of determining, keeping, and distributing the time, calls for the first attention.
Entering the transit room, the first thing that strikes the visitor is the extreme solidity with which the great telescope is mounted. It turns but in one plane, that of 'longitude nought,' and its pivots are supported by the pair of great stone pillars which we have already spoken of as occupying the principal part of the transit-room area, and the foundations of which go deep down under the surface of the hill. On the west side of the telescope, and rigidly connected with it, is a large wheel some six feet in diameter, and with a number of wooden handles attached to it, resembling the steering-wheel of a large steamer. This wheel carries the setting circle, which is engraved upon a band of silver let into its face near its circumference, a similar circle being at the back of the wheel nearer the pillar. Eleven microscopes, of which only seven are ordinarily used, penetrate through the pier, and are directed on to this second circle.
The present transit is the fourth which the Observatory has possessed, and its three predecessors, known as Halley's, Bradley's, and Troughton's, respectively, are still preserved and hang on the walls of the transit room, affording by their comparison an interesting object-lesson in the evolution of a modern astronomical instrument.
The watcher who wishes to observe the passing of a star must note two things: he must know in what direction to point his telescope, and at what time to look for the star. Then, about two minutes before the appointed time, he takes his place at the eyepiece. As he looks in he sees a number of vertical[157] lines across his field of view. These are spider-threads placed in the focus of the eye-piece. Presently, as he looks, a bright point of silver light, often surrounded by little flashing, vibrating rays of colour, comes moving quickly, steadily onward—'swims into his ken,' as the poet has it. The watcher's hand seeks the side of the telescope till his finger finds a little button, over which it poises itself to strike. On comes the star, 'without haste, without rest,' till it reaches one of the gleaming threads. Tap! The watcher's finger falls sharply on the button. Some three or four seconds later and the star has reached another 'wire,' as the spider-threads are commonly called. Tap! Again the button is struck. Another brief interval and the third wire is reached, and so on, until ten wires have been passed, and the transit is over. The intervals are not, however, all the same, the ten wires being grouped into three sets, two of three apiece, and the third of four.
Each tap of the observer's finger completed for an instant an electric circuit, and recorded a mark on the 'chronograph.' This is a large metal cylinder covered with paper, and turned by a carefully-regulated clock once in every two minutes. Once in every two seconds a similar mark was made by a current sent by means of the standard sidereal clock of the Observatory. The paper cover of the chronograph after an hour's work shows a spiral trace of little dots encircling it some thirty times. These dots are at regular intervals, about an inch apart, and are the marks made by the clock. Interspersed between them are certain other dots, in sets of ten; and these[158] are the signals sent from the telescope by the transit observer. If, then, one of the clock dots and one of the observer's dots come exactly side by side, we[159] know that the star was on one of the wires at a given precise second. If the observer's dot comes between two clock dots, it is easy, by measuring its distance from them with a divided scale, to tell the instant the star was on the wire to the tenth of a second, or even to a smaller fraction. Whilst, since the transit was taken over ten wires, and the distance of each wire from the centre of the field of view is known, we have practically ten separate observations, and the average of these will give a much better determination of the time of transit than a single one would.
But let the watcher be ever so little too slow in setting his telescope, or ever so little late in placing himself at his eye-piece, and the star will have passed the wire, and as it smoothly, resistlessly moves on its inexorable way, will tell the tardy watcher in a language there is no mistaking, 'Lost moments can never be recalled.' The opportunity let slip, not until twenty-four hours have gone by will another chance come of observing that same star.
It is the stars that are chiefly used in this determination, partly because the stars are so many, whilst there is but one sun. If, therefore, clouds cover the sun at the important moment of transit, the astronomer may well exclaim, so far as this observation is concerned, 'I have lost a day!' The chance will not be offered him again until the following noon. But if one star is lost by cloud, there are many others, and the chance is by no means utterly gone. Beside, the sun enables us to tell the time only at noon; the stars enable us to find it at various times throughout the entire night; indeed, throughout both day and[160] night, since the brighter stars can be observed in a large telescope even during the day.
There are two great standard clocks at the Observatory: the mean solar clock and the sidereal clock. The latter registers twenty-four hours in the precise time that the earth rotates on its axis. A 'day' in our ordinary use of the term is somewhat longer than this; it is the average time from one noon to the next, and as the earth whilst turning round on its axis is also travelling round the sun, it has to rather more than complete a rotation in order to bring the sun again on to the same meridian. A solar day is therefore some four minutes longer than an actual rotation of the earth, i.e. a sidereal day, as it is called, since such rotation brings a star back again to the same meridian.
The sidereal clock can therefore be readily checked by the observation of star transits, for the time when the star ought to be on the meridian is known. If, therefore, the comparison of the transit taps on the chronograph with the taps of the sidereal clock show that the clock was not indicating this time at the instant of the transit, we know the clock must be so much fast or slow. Similarly, the difference which should be shown between the sidereal and solar clocks at any moment is known; and hence when the error of the sidereal clock is known, that of the solar can be readily found.
It is often quite sufficient to know how much a clock is wrong without actually setting its hands right; but it is not possible to treat the Greenwich clock so, for it controls a number of other clocks[161] continually, and sends hourly signals out over the whole country, by which the clocks and watches all over the kingdom are set right.
In the lower computing room, below the south window, we find the Time-Desk, the head-quarters of the Time Department. This is a very convenient place for the department, since one of the chronometer rooms, formerly Bradley's transit room, opens out of the lower computing room; the transit instrument is just beyond; it is close to the main gate of the Observatory, and so convenient for chronometer makers or naval officers bringing chronometers or coming for them, whilst just across the courtyard is the chronograph room, with the Battery Basement, in which the batteries for the electric currents are kept, and the Mean Solar Clock lobby, with the winch for the winding of the time-ball at the head of the stairs above it. These rooms do not exhaust the territory of the department, since it owns two other chronometer rooms on the ground floor and first floor respectively of the S.-E. tower.
At the time-desk means are provided for setting the clock right very easily and exactly. Just above the desk are a range of little dials and bright brass knobs, that almost suggest the stops of a great organ.
Two of these little dials are clock faces, electrically connected with the solar and sidereal standard clocks, so that, though these clocks are themselves a good way off, in entirely different parts of the Observatory, the time superintendent, seated here at the time-desk, can see at once what they are indicating.[162] Between the two is a dial labelled 'Commutator.' From this dial a little handle usually hangs vertically downwards, but it can be turned either to the right or to the left, and when thus switched hard over, an electric current is sent through to the mean solar clock. If now we leave the computing room and cross the courtyard to the extreme north-west corner, we find the Mean Solar Clock in a little lobby, carefully guarded by double doors and double windows against rapid changes of temperature. Opening the door of the clock case, we see that the pendulum carries on its side a long steel bar, and that this bar as the pendulum swings passes just over the upper end of an electro-magnet. When the current is switched on at the commutator, this electro-magnet attracts or repels the steel bar according to the direction of the current, and the action of the clock is accordingly quickened or retarded. To put the commutator in action for one minute will alter the clock by the tenth of a second. As the error of the clock is determined twice a day, shortly before ten o'clock in the morning, and shortly before one o'clock in the afternoon, its error is always small, usually only one or two tenths. These two times are chosen because, though time-signals are sent over the metropolitan area every hour from the Greenwich clock through the medium of the Post Office, at ten and at one o'clock signals are also sent to all the great provincial centres. Further, at one o'clock the time balls at Greenwich and at Deal are dropped, so that the captains of ships in the docks, on the river, or in the Downs may check their chronometers.
The Time-Ball is dropped directly by the mean solar clock itself. It is raised by means of a windlass turned by hand-power to the top of its mast just before one o'clock. Connected with it is a piston working in a stout cylinder. When the ball has reached the top of the mast, the piston is lightly supported by a pair of catches. These catches are pulled back by the hourly signal current, and the piston at once falls sharply, bringing the ball with it. But after a fall of a few feet, the air compressed by the piston acts as a cushion and checks the fall, the ball then gently and slowly finishing its descent. The instant of the beginning of the fall is, of course, the true moment to be noted.
The other dials on the time-desk are for various purposes connected with the signals. One little needle in a continual state of agitation shows that the electric current connecting the various sympathetic clocks of the Observatory is in full action. Another receives a return signal from various places after the despatch of the time-signal from Greenwich, and shows that the signal has been properly received at the distant station, whilst all the many electric wires within the Observatory or radiating from it are made to pass through the great key-board, where they can be at once tested, disconnected, or joined up, as may be required.
The distribution of Greenwich time over the island in this way is thus a simple matter. The far more important one of the distribution of Greenwich time to ships at sea is more difficult. The difficulty lay in the construction of a clock or watch, the rate[164] of which would not be altered by the uneasy motion of a ship, or by the changes of temperature which are inevitable on a voyage. Two hundred years ago it was not deemed possible to construct a watch of anything like sufficient accuracy. They would not even keep going whilst they were being wound, and would lose or gain as much as a minute in the day for a fall or rise of 10° in temperature. This was owing to the extreme sensitiveness of the balance spring—which takes the place in a watch of a pendulum in a clock—to the effects of temperature. The British Government, therefore, in 1714 offered a[165] prize of the amount of £20,000 for a means of finding the longitude at sea within half a degree, or, in other words, for a watch that would keep Greenwich time correct to two minutes in a voyage across the Atlantic. In 1735, James Harrison, the son of a Yorkshire carpenter, succeeded in solving the problem. His method was to attach a sort of automatic regulator to the spring which should push the regulator over in one direction as the temperature rose, and bring it back as it fell. This he effected by fastening together two strips of brass and steel. The brass expanded with heat more rapidly than the steel, and hence with a rise of temperature the strip[166] bent over on the steel side. This was the first germ of the idea of making watches 'compensated for temperature;' watches, that is, which maintain practically the same rate whether they are in heat or cold, an idea now brought to great perfection in the modern chronometer.
The great reward the Government had offered stimulated many men to endeavour to solve the problem. Of these, Dr. Halley, the second Astronomer Royal, and Graham, the inventor of the astronomical clock, were the most celebrated. But when Harrison, then poor and unknown, came to London in 1735, and laid his invention before them, with an utter absence of self-seeking, and in the true scientific spirit, they gave him every assistance.
Harrison's first four time-keepers are still preserved at the Royal Observatory. He did not, however, receive his reward until a facsimile of the fourth had been made by his apprentice, Larcum Kendall. The latter is preserved at the Royal Observatory. There is a Larcum Kendall at the Royal Institution which is said to have been used by Captain Cook. Harrison's chronometer was sent on a trial voyage to Jamaica in 1761, and on its return to Portsmouth in the following year it was found that its complete variation was under the two minutes for which the Government had stipulated.
Since Harrison's day the improvement of the chronometer has been carried on almost to perfection, and now the care and rating of chronometers for the Royal Navy is one of the most important duties of the Observatory.
A visitor who should make the attempt to compare a single chronometer with a standard clock would probably feel very disheartened when, after many minutes of comparison, he had got out its error to the nearest second, were he told that it was his duty to compare the entire army here collected, some five hundred or more, and to do it not to the second, but to the nearest tenth of a second. Practice and system make, however, the impossible easy, and one assistant will quietly walk round the room calling out the error of each chronometer as he passes it, as fast as a second assistant seated at the table can enter it at his dictation in the chronometer ledgers. The seconds beat of a clock sympathetic with the solar standard, rings out loud and clear above the insect-like chatter of the ticking of the hundreds of chronometers, and wherever the assistant stands, he has but to lift his eyes to see straight before him, if not a complete clock-face, at least a seconds dial moving in exact accordance with the solar standard.
The test to which chronometers are subjected is not merely one of rate, but one of rate under carefully altered conditions. Thus they may be tried with the XII pointing in succession to the four points of the compass, or, in the case of chronometer watches, they may be laid flat down on the table or hung from the ring or pendant, or with the ring right or left, as it would be likely to be when carried in the waistcoat pocket. But the chief test is the performance of a chronometer when subjected to considerable heat for a long period. This is a matter of[170] great consequence, since a chronometer travelling from England to India, Australia, or the Cape, would necessarily be subjected to very different conditions of temperature from those to which it would be exposed in England. They are therefore kept for eight weeks in a closed stove at a temperature of about 85° or 90°. At one time a cold test was also applied, and Sir George Airy, the late Astronomer Royal, in one of his popular lectures, drew a humorous comparison between the unhappy chronometers thus doomed to trial, now in heat and now in frost, and the lost spirits whom Dante describes as alternately plunged in flame and ice. The cold test has, however, been done away with. It is perfectly easy on the modern ship to keep the chronometer comfortably warm even on an Arctic expedition. The elaborate cold testing applied to Sir George Nares' chronometers before he started on his polar journey was found to have been practically quite superfluous; the chronometers were, if anything, kept rather too warm. The exposure of the chronometer in the cooling box, moreover, was found to be attended with a risk of rusting its springs.
Once the determination of the longitude at sea became possible, it was clearly the next duty to fix with precision the position of the principal places, cities, ports, capes, islands, the world over. Of all the work done in this department none has ever been done better, in proportion to the means at command, than that accomplished by Captain Cook in his celebrated three voyages. As has already been pointed out, it is the extent and thoroughness of the hydrographic
[173] surveys of the British Admiralty which have largely contributed to the honour done to England by the international selection of the English meridian, and of English standard time, as in principle those for the whole civilized world. The generosity and public spirit therefore which led the second Astronomer Royal to help forward and support his rival, has almost directly led to this great distinction accruing to the Observatory of which he was the head.
Three different methods have successively been used in the determination of longitudes of distant places. In each case the problem required was to ascertain the time at the standard place, say Greenwich, at the same time that it was being determined in the ordinary way at the given station. One method of ascertaining Greenwich time when at a distance from it was, as stated in Chapter I., to use the moon, as it were, as the hand of a vast clock, of which the sky was the face and the stars the dial figures. This is the method of 'lunar distances,' the distances of the moon from a certain number of bright stars being given in the Nautical Almanac for every three hours of Greenwich time.
As chronometers were brought to a greater point of perfection, it was found easier and better in many cases to use 'chronometer runs,' that is, to carry backwards and forwards between the two stations a number of good chronometers, and by constant comparison and re-comparison to get over the errors which might attach to any one of them.
But of late years another method has proved available. Distant nations are now woven together[174] across thousands of miles of ocean by the submarine telegraph. The American reads in his morning paper a summary of the debates of the previous night in the House of Commons at Westminster. The Londoner watches with interest the scores of the English cricket team in Australia. It is now therefore possible for an astronomer in England to record, should he so desire, the time of the transit of a star across the wires of his instrument, not only on his own chronograph, but upon that of another observatory, it may be 2000 miles away. Or, much more conveniently, each observer may independently determine the error of his own clock, and then bring his[175] clock into the current, so that it may send a signal to the chronograph of the other station.
In one way or another this work of the determination of geographical longitudes has been an important part of the extra-routine work at Greenwich, part of the work which has built up and sustained its claim to define 'longitude nought'; and many distinguished astronomers, especially from the leading observatories of the Continent, have come here from time to time to obtain more accurately the longitude of their own cities. The traces of their visits may be seen here and there about the Observatory grounds in flat stones which lie level with the surface, and bear a name and date like the gravestones in some old country churchyard. These are not, as one might suppose, to mark the burial-places of deceased astronomers, but record the sites where, on their visits for longitude purposes, different foreign astronomers have set up their transit instruments. Now, however, a permanent pier has been erected in the courtyard, and a neat house—the Transit Pavilion—built over it, so that in all probability no fresh additions will be made to these sepulchral-looking little monuments.
It might be asked, What reason is there for a foreign observer to come over to England for such a purpose? Would it not be sufficient for the clock signals to be exchanged? But a curious little fact has come out with the increase of accuracy of transit observation, and that is, that each observer has his own particular habit or method of observation. A hundred years ago, Maskelyne, the fifth Astronomer[176] Royal, was greatly disturbed to find that his assistant, David Kinnebrook, constantly and regularly observed a star-transit a little later than he did himself. The offender was scolded, warned, exhorted, and finally, when all proved useless to bring his observations into exact agreement with the Astronomer Royal's, dismissed as an incompetent observer. As a matter of fact, poor Kinnebrook has a right to be regarded as one of the martyrs of science, and Maskelyne, by this most natural but mistaken judgment, missed the chance of making an important discovery, which was not made until some thirty years later. Astronomers now would be more cautious of concluding that observations were bad simply because they differed from what had been expected. They have learnt by experience that these unexpected differences are the most likely hunting-ground in which to look for new discoveries.
In a modern transit observation with the use of the chronograph it will be seen at once that before the observer can register a star-transit on the chronograph, he has to perceive with his eye that the star has reached the wire, he has to mentally recognize the fact, and consciously or unconsciously to exert the effort of will necessary to bring his finger down on the button. A very slight knowledge of character will show that this will require different periods of time for different people. It will be but a fraction of a second in any case, but there will be a distinct difference, a constant difference, between the eager, quick, impulsive man who habitually anticipates, as it were, the instant when he sees star and wire[177] together, and the phlegmatic, slow-and-sure man who carefully waits till he is quite sure that the contact has taken place, and then deliberately and firmly records it. These differences are so truly personal to the observer that it is quite possible to correct for them, and after a given observer's habit has become known, to reduce his transit times to those of some standard observer. It must, of course, be remembered that this 'personal equation' is an exceedingly minute quantity, and in most cases is rather a question of hundredths of seconds than of tenths.
It will be seen from the foregoing description how little of what may be termed the picturesque or sensational side of astronomy enters into the routine of the Time Department, the most important of all the departments of the Observatory. The daily observation of sun and of many stars—selected from a carefully chosen list of some hundreds, and known as 'clock stars'—the determination of the error of the standard clock to the hundredth of a second if possible, and its correction twice a day, the sending out of time signals to the General Post Office and other places, whence they are distributed all over the country; the care, winding, and rating of hundreds of chronometers and chronometer watches, and from time to time the determination of the longitude of foreign or colonial cities, make up a heavy, ceaseless routine in which there is little opportunity for the realization of an astronomer's life as it is apt to be popularly conceived.
Yet there is interest enough in the work. There[178] is the charm which always attaches to work of precision, the delight of using delicate and exact instruments, and of obtaining results of steadily increasing perfection. It may be akin to the sporting passion for record-breaking, but surely it is a noble form of it which has led the assistants, in recent years, to steadily increase the number of observations in a normal night's work up to the very limit, taking care the while that their accuracy has in no degree suffered. In longitude work also 'the better is the enemy of the good,' and there is the ambition that each fresh determination shall be markedly more precise than all that have preceded it. The constant care of chronometers soon reveals a kind of individuality in them which forms a fresh source of interest, whilst if a man has but a spark of imagination, how easily he will wrap them round with a halo of romance!
Glance through the ledgers, and you will see how some of them have heard the guns at the siege of Alexandria, others have been carried far into the frozen north, others have wandered with Livingstone or Cameron in the trackless forests of equatorial Africa.
More striking still are those pages across which the closing line has been drawn; never again will the time-keeper there scheduled return to the kindly inquisition of Flamsteed Hill. This sailed away in the Wasp, and was swallowed up in the eastern typhoon; that went down in the sudden squall that smote the Eurydice off the Isle of Wight; these foundered with the Captain. The last fatal journey[179] of Sir John Franklin to find the North-West Passage leaves its record here; the chronometers of the Erebus and Terror will never again appear on the Greenwich muster roll. Land exploration claims its victims too. Sturt's ill-fated expedition across Australia, and Livingstone's last wandering, are represented.
Sometimes an amusing entry interrupts the silent pathos of these closed pages. 'Lost by Mr. Smith on the coast of Africa,' reads at first sight like a rather thin attempt of some one to shift the responsibility of his own carelessness on to the broad shoulders of Mr. Nobody. In reality it probably gives a hint of the necessary, dangerous, and exciting work of slave-dhow chasing which gives employment[180] to our ships on the African coast. 'Mr. Smith' was no doubt a petty officer who was told off to carry the chronometer for a boat's crew sent to search for a slave-dhow up some equatorial estuary. Probably the dhow was found, and the Arabs who manned it gave so stout a resistance that 'Mr. Smith' and his men had other things to do than take care of chronometers before they could overcome them. We may take it that the real story outlined here was one of courage and hard fighting, not of carelessness and shirking.
Stories of higher valour and nobler courage yet are also hinted: the calm discipline of the crew of the Victoria as she sank from the ram of the Camperdown, the yet nobler devotion of the men of the Birkenhead, as they formed up in line on deck and cheered the boats that bore away the women and children to safety, whilst they themselves went down with the ship into the shark-crowded sea.
CHAPTER VII
THE TRANSIT AND CIRCLE DEPARTMENTS
The determination of time is a duty the importance of which readily commends itself to the general public. It is easy to see that in any civilized country it is very necessary to have an accurate standard of time. Our railways and telegraphs make it quite impossible for us to be content with the rough-and-ready sun-dial which satisfied our forefathers. But it should be remembered that it was neither to establish a 'longitude nought,' nor to create a system of standard time, that Greenwich Observatory was founded in 1675. It was for 'The Rectifying the Tables of the Motions of the Heavens and the Places of the Fixed Stars, in order to find out the so-much-desired Longitude at Sea for the perfecting the Art of Navigation.'
The two related departments, therefore, those of the Transit and the Circle, which are concerned in the work of making star-catalogues, come next in order to the Time Department. Though both departments deal with the same instrument, the transit circle, they are at present placed at opposite ends of the Observatory domain; the Circle Department being lodged in the upper computing room[182] of the old building; the Transit Department in the south wing of the New Observatory in the south ground.
It may be asked why, if this were the purpose of the Observatory at its foundation, two and a quarter centuries ago; if, as was the case, the work was set on foot from the beginning and was carried out with every possible care, how comes it that it is still the fundamental work of the Observatory, and, instead of being completed, has assumed greater proportions at the present day than ever before?
The answer to this inquiry may be found in a special application of the old proverb, originally directed against the discontent of man: 'The more he has, the more he wants.' For, however paradoxical it may seem, it is true that the fuller a star-catalogue is, and the more accurate the places of the stars that it contains, the greater is the need for a yet fuller catalogue, with places more accurate still.
It is worth while following up this paradox in some detail, as it affords a very instructive example of the way in which a work started on purely utilitarian grounds extends itself till it crosses the undefined boundary and enters the region of pure science.
We have no idea who made the earliest census of the sky. It is written for us in no book; it is not even engraved on any monument. And yet no small portion of it is in our hands to-day, and, strangest of all, we are able to fix fairly closely the time at which it was made, and the latitude in which its compiler lived. The catalogue is very unlike our star-catalogues of to-day. The places of the stars are very[183] roughly indicated; and yet this catalogue has left a more enduring mark than all those that have succeeded it. The catalogue simply consists of the star names.
An old lady who had attended a University Extension lecture on astronomy was heard to exclaim: 'What wonderful men these astronomers are! I can understand how they can find out how far off the stars are, how big they are, and what they weigh—that is all easy enough; and I think I can see how they find out what they are made of. But there is one thing that I can't understand—I don't know how they can find out what are their names!' This same difficulty, though with a much deeper meaning than the old lady in her simplicity was able to grasp, has occurred to many students of astronomy. Many have wished to know what was the meaning of, and whence were derived, the sonorous names which are found attached to all the brighter stars on our celestial globes: Adhara, Alderamin, Betelgeuse, Denebola, Schedar, Zubeneschamal, and many more. The explanation lies here. Some 5000 years ago, a man, or college of men, living in latitude 40° north, in order that they might better remember the stars, associated certain groups of them with certain fancied figures, and the individual star names are simply Arabic words designed to indicate whereabouts in its peculiar figure or constellation that special star was situated. Thus Adhara means 'back,' and is the name of the bright star in the back of the great Dog. Alderamin means 'right arm,' and is the brightest star in the right arm of Cepheus, the king.[184] Betelgeuse is 'giant's shoulder,' the giant being Orion; Denebola is 'lion's tail.' Schedar is the star on the 'breast' of Cassiopeia, and Zubeneschamal is 'northern claw,' that is, of the Scorpion. So far is clear enough. The names of the stars for the most part explain themselves; but whence the constellations derived their names, how it was that so many snakes and fishes and centaurs were pictured out in the sky, is a much more difficult problem, and one which does not concern us here.
One point, however, these old constellations do tell us, and tell us plainly. They show that the axis of the earth, which, as the earth travels round the sun, moves parallel with itself, yet, in the course of ages, itself rotates so as in a period of some 26,000 years to trace out a circle amongst the stars. This is the cause of what is called 'precession,' and explains how it is that the star we call the pole-star to-day was not always the pole-star, nor will always remain so. We learn this fact from the circumstance that the old constellations do not cover the entire celestial sphere. They leave a great circular space of 40° radius unmapped in the southern heavens. This simply means that the originators of the constellations lived in 40° north latitude, and stars within 40° of their south pole never rose above their horizon, and consequently were never seen, and could not be mapped, by them. In like manner, the star census taken at Greenwich Observatory does not include the whole sky, but leaves a space some 52° in radius round our south pole. Since the latitude of Greenwich is nearly 52° north, stars within that distance of[185] the south pole do not rise above our horizon, and are never seen here. But if we compare the vacant space left by the old original constellations with the vacant space left by a Greenwich catalogue of to-day, we see that the centre of the first space, which must have been the south pole of that time, is a long way from the centre of the second space—our south pole of to-day. The difference tells us how far the pole has moved since those old forgotten astronomers did their work. We know the rate at which the pole appears to move, by comparing our more modern catalogues one with another; and so we are able to fix pretty nearly the time when lived those old first census-takers of the stars, whose names have perished so completely, but whose work has proved so immortal.
These old workers gave us the constellation groupings and names which still remain to us, and are still in common, every-day use. Their work affords us the most striking illustration of the result of precession, but precession itself was not recognized till nearly 3000 years after their day, when a marvellous genius, Hipparchus, established the fact, and 'built himself an everlasting name' by the creation of a catalogue of over 1000 stars prepared on modern principles. That catalogue formed the basis of one which survives to us at the present time, and was made some 1750 years ago by Claudius Ptolemy, the great astronomer of Alexandria, whose work, which still bears the proud name of Almagest, 'The Greatest,' remained for fourteen centuries the one universal astronomical text-book.
A modern catalogue contains, like that of Ptolemy, four columns of entry. The first gives the star's designation; the second an indication of its brightness; the third and fourth the determinations of its place. These are expressed in two directions, which, in modern catalogues, not in Ptolemy's, correspond on the celestial sphere to longitude and latitude on the terrestrial. Distance north or south of the celestial equator is termed 'declination,' corresponding to terrestrial latitude. Distance in a direction parallel to the equator is termed 'right ascension,' corresponding to terrestrial longitude. For geographical purposes we conceive the earth to be encircled by two imaginary lines at right angles to each other—the one, the equator, marked out for us by the earth itself; the other, 'longitude nought,' the meridian of Greenwich, fixed for us by general consent, after the lapse of centuries, by a kind of historical evolution. On the celestial globe in like manner we have two fundamental lines—one, the celestial equator, marked out for us by nature; the other at right angles to it, and passing through the poles of the sky, adopted as a matter of convenience. But a difficulty at once confronts us—Where can we fix our 'right ascension nought'? What star has the right to be considered the Greenwich of the sky?
The difficulty is met in the following manner: For six months of the year, the summer months, the sun is north of the celestial equator; for the other six months of the year, the months of winter, it is south of it. It crosses the equator, therefore, twice in the year—once when moving northward at the[187] spring equinox; once when moving southward at the equinox of autumn. The point where it crosses the equator at the first of these times is taken as the fundamental point of the heavens, and the first sign of the zodiac, Aries the Ram, is said to begin here, and it is called, therefore, 'the first point of Aries.'
One of the very first facts noticed in the very early days of astronomy was that, as the stars seemed to move across the sky night by night, they seemed to move in one solid piece, as if they were lamps rigidly fixed in one and the same solid vault. Of course it has long been perfectly understood that this apparent movement was not in the least due to any motion of the stars, but simply to the rotation of the earth on its axis. This rotation is the smoothest, most constant, and regular movement of which we know. It follows, therefore, that the interval of time between the passage of one star across the meridian of Greenwich and that of any other given star is always the same. This interval of time is simply the difference of their right ascension. If we are able, then, to turn our transit instrument to the sun, and to a number of stars, each in its proper turn, and by pressing the tapping-piece on the instrument as the sun or star comes up to each of the ten wires in succession, to record the times of its transit on the chronograph, we shall have practically determined their right ascensions—one of the two elements of their places.
The other element, that of declination, is found in a different manner. The celestial equator, like the terrestrial, is 90° from the pole. The bright star[188] Polaris is not exactly at the north pole, but describes a small circle round it. Twice in the twenty-four hours it transits across the meridian—once when going from east to west it passes above the pole, once when going from west to east below the pole. The mean between these two altitudes of Polaris above the horizon gives the position of the true pole.
A complete transit observation of a star consists therefore of two operations. The observer, as we have already described, sees a star entering the field of the telescope, and as it swims forward, he presses the galvanic button, which sends a signal to the chronograph as the star comes up to each of the ten vertical wires in succession. But, beside the ten wires, there are others. Two vertical wires lie outside the ten of which we have already spoken, and there is also a horizontal wire. The latter can be moved by a graduated screw-head just above the eye-piece, and as the star comes in succession to these two vertical wires, this horizontal wire is moved by the screw-head, so as to meet the star at the moment it is crossing the vertical wire, and the observer presses a second little button, which records the position of the horizontal wire on a small paper-covered drum. Then, the transit over, the observer leaves the telescope and comes round to the outside of the west pier. Here he finds seven large microscopes, which pierce the whole thickness of the pier, and are directed towards the circumference of a large wheel which is rigidly attached to the telescope and revolves with it. This wheel is six feet in diameter, and has a silver
[191] circle upon both faces. Each circle is divided extremely carefully into 4320 divisions—these divisions, therefore, being about the one-twentieth of an inch apart. There are, therefore, twelve divisions to every degree (12 × 360 = 4320), and each division equals five minutes of arc. The lowest microscope is the least powerful, and shows a large part of the circle, enabling the observer to see at once to what degree and division of a degree the microscope is pointing. The other six microscopes are very carefully placed 60° apart—as equally placed as they possibly can be. These microscopes are all fitted with movable wires—wires moved by a very fine and delicate screw; the screw-head having divisions upon it so that the exact amount of its movement can be told. Each of the six screw-heads will read to the one five-thousandth part of a division of the circle; in other words, to the one hundred thousandth part of an inch. Using all six microscopes, and taking their mean, we are able to read to the one-hundredth of a second of arc. If, therefore, the observations could be made with perfect certainty down to the extremest nicety of reading which the instrument supplies, we should be able to read the declination of a star to this degree of refinement. It may be added that a halfpenny, at a distance of three miles, appears to be one second of arc in diameter; at three hundred miles it would be one-hundredth of a second. It need scarcely be said that we cannot observe with quite such refinement of exactness as this would indicate. Nevertheless, this exactness is one after which the observer is constantly striving, and tenths,[192] even hundredths, of seconds of arc are quantities which the astronomer cannot now neglect.
The observer has then to read the heads of all these seven microscopes on the pier side, and also two positions of the horizontal wire on the screw-head at the eye-piece. The following morning he will also read off from the chronograph-sheet the times when he made the ten taps as the star passed each of the ten vertical wires. There are, therefore, nine entries to make for one position of a star in declination, and ten for one position of a star in right ascension. The observer will also have to read the barometer to get the pressure of the air at the time of observation, and one thermometer inside the transit room, and another outside, to get the temperature of the air. In some cases thermometers at different heights in the room are also read. A complete observation of a single star means, therefore, the entry of two-and-twenty different numbers.
It may be asked, What is the use of reading the barometer and thermometer? The answer to the question can only be given by contradicting a statement made above, that the true pole lay midway between the position of the telescope when pointing to the pole-star at its upper transit, and its position when pointing to it at its lower transit. The pole being very high in the heavens in this country, there are a great number of stars that, like the pole-star, cross the meridian twice in the twenty-four hours—once when they pass above the pole, moving from east to west, once when they pass below it, moving from west to east As the real distance of a star[193] from the true pole does not alter, it follows that we ought to get the position of the pole from the mean of the two transits of any of these stars, and they ought all to exactly agree with each other. But they do not. So, too, I said that the stars all appeared to move as in a single piece. If, then, we constructed an instrument with its axis parallel to the axis of the earth, and fixed a telescope to it, pointing to any particular star, if we turn the telescope round as fast from east to west as the earth itself is turning from west to east—if we built an equatorial, that is to say—we ought to find that the star once in the centre of the field would remain there. As a matter of fact, when the star got near the horizon it would soon be a long way from the centre of the field.
Sir George Airy, the seventh Astronomer Royal, makes, with reference to this very point, the following remarks:
'Perhaps you may be surprised to hear me say the rule is established as true, and yet there is a departure from it. This is the way we go on in science, as in everything else; we have to make out that something is true, then we find out under certain circumstances that it is not quite true; and then we have to consider and find out how the departure can be explained.'
In this particular case, the disturbing cause is found in the action of our own atmosphere. The rays of light from the star are bent out of a perfectly straight course as they pass through the various layers of that atmosphere, layers which necessarily become denser the closer we get to the actual surface of the earth. Every celestial body therefore appears[194] to be a little higher in the sky than it really is. This action is most noticeable at the horizon, where it amounts to about half a degree. As both sun and moon are about half a degree in diameter, it follows that when they have really just entirely sunk below the horizon they appear to be just entirely above it. It happens, in consequence, on rare occasions, that an eclipse of the moon will take place when both sun and moon are together seen above the horizon.
It was a great matter to discover this effect of refraction. It was soon seen that it was not constant, that it varied with both temperature and pressure. It is, indeed, the most troublesome of all the hindrances to exact observation with which the astronomer has to contend; partly because of its large amount—half a degree, as has been already said, in the extreme case—and partly because it is difficult in many cases to determine its exact effect.
The double observation with the transit circle gives us, then, the place in the sky where the star appeared to be at the moment of observation, not its true place; to find that true place we have to calculate how much refraction had displaced the star at the particular height in the sky, and at the particular temperature and atmospheric pressure at which the observation was made.
The transit circle is a comparatively recent instrument. In earlier times the two observations of right ascension and declination were entrusted to perfectly separate instruments. The transit instrument was mounted as the transit circle is, between two solid stone piers, and moved in precisely the same way.[195] But the great six-foot wheel, which was made as stiff as it possibly could be, was mounted on the face of a great stone pier or wall, from which circumstance it was called the 'mural circle,' and a light telescope was attached to it which turned about its centre. This arrangement had a double disadvantage—that the two observations had to be made separately, and[196] the mural circle, not being a symmetrical instrument, was liable to small errors which it was difficult to detect. Thus, being supported on one side only, a flexure or bending outwards of either telescope or circle, or both, might be feared.
It was for this reason that Pond set up a pair of mural circles, one on the east side of its supporting pier and the other on the west.[3] His plan was not only to have each star observed simultaneously in the two instruments, a plan by which, at the cost of some additional labour, he would have got rid, to a large extent, of the individual errors of the two separate instruments, inasmuch as, on the whole, it might have been expected that the errors of the two instruments would have been very nearly equal in amount, but of opposite character. The differences, too, between the two instruments would have afforded the means for tracing these small errors to their respective causes, and so ascertaining the laws to which they were subject.
Pond went further still. He added to the mural circle a simple instrument, the extreme value of which every astronomer recognizes to-day—the mercury trough. Not only was the star to be observed by both circles when the two telescopes were pointing directly to it, it was also to be observed by reflection; the telescopes were to be pointed down towards a basin of mercury, in which the image of the star would be seen reflected. The mercury being a[197] liquid, its surface is perfectly horizontal; and, since the law of reflection is that the angle of incidence is equal to the angle of reflection, it follows that the telescope, when pointed down toward the mercury trough, points just at as great an angle below the horizon as, when it is set directly on the star, it points above it. If the circle, therefore, be carefully read at both settings, half the difference between the two readings will give the angular elevation of the star above the horizon. A combination, therefore, of all four observations, that is to say, one reflection and one direct with each of the telescopes, would give an exceedingly exact value for the star's altitude. The conception of this method gives a striking idea of Pond's thoroughness and skill as a practical observer, and it is a distinct blot upon Airy's justly high reputation in the same line that he discontinued the system upon his accession to office.
However, in 1851, as already mentioned, Airy substituted for the two separate instruments—the transit and mural circle—the transit circle, which, unlike the mural circle, is equally supported on both sides. This, however, does not free it from the liability to some minute flexure in the direction of its length, from the weight of its two ends, and the mercury trough is used for the detection of such bending, should it exist. The present practice is to observe a star both by reflection and directly in the course of the same transit. The observer sets the telescope carefully before ever the star comes into the field of view, and reads his seven microscopes. Then he climbs up a narrow wooden staircase and watches[198] the star transit nearly half across the field. Then comes a rush, the observer swings himself down the ladder, unclamps the telescope, turns it rapidly up to the star itself, clamps it again, flings himself on his back on a bench below the telescope, and does it so quickly that he is able to observe the star across the second half of the field. There is no time for dawdling, no room for making any mistakes; the stars never forgive; 'they haste not, they rest not;' and if the unfortunate observer is too slow, or makes some slip in his second setting, the star, cold and inexorable, takes no pity, and moves regardless on.
It will be seen that a considerable amount of work is involved in taking a single observation of a star-place. But in making a star-catalogue it is always deemed necessary to obtain at least three observations of each star; and many are observed much more frequently.
A modern star-catalogue contains, like Ptolemy's, four columns. It contains also several more. Of these the principal are devoted to the effect of precession. As precession is caused by a movement of the earth's axis making the pole of the sky seem to describe a circle in the heavens, it follows that the celestial poles, and the celestial equator with them are slowly, but continually, changing their place with respect to the stars, and therefore that the declinations of the stars are always undergoing change, and as the equator changes, the point where the sun crosses it in spring—the first point of Aries—changes also, and with it the stars' right ascensions.
To make one determination of a star's place[199] comparable with another made at another time, it is clear that we must correct for the effects of precession in the interval of time between the two observations, and for the effects of refraction. But observations made with the transit circle must also be corrected for errors in the instrument itself. The astronomer will see to it that his instrument is made and is set up as perfectly as possible. The pivots on which it turns must be exactly on the same level; they must point exactly east and west, and the axis of the telescope must be exactly at right angles to the line joining the pivots in all positions of the instrument. These conditions are very nearly fulfilled, but never absolutely. Day by day, therefore, the astronomer has to ascertain just how much his instrument is in error in each of these three matters. Were his instrument absolutely without error to-day, he could not assume that it would remain so, nor, if he had measured the amount of its errors yesterday, would it be safe to assume that those errors would not change to-day.
In the examination of these sources of error the mercury trough comes again into use. The transit circle is turned directly downwards, and the mercury trough brought below it. A light is so arranged as to illuminate the field of the telescope, and the observer, looking in, sees the ten transit wires and the one declination wire, and also sees their images reflected from the surface of the mercury. If the telescope be pointing exactly down towards the surface of the mercury, then the image of the declination wire will fall exactly on the declination wire itself,[200] and by reading the circle we can tell where the zenith point of the circle is. Similarly, if the pivots of the telescope are precisely on the same level, the centre wire of the right ascension series would coincide with its reflected image. A third point is determined by looking through the eye-piece of the north collimator telescope—that is to say, the telescope mounted in a horizontal position at the north end of the room—at the spider lines in the focus of the south collimator. In order to get this view, the transit telescope has either to be lifted up out of its usual position, or else the middle of the tube has to be opened. The spider lines in the north collimator are then made to coincide with the image of the wires of the south collimator. The transit telescope is then turned first to one collimator, then to the other, and the central wire of the right ascension series is turned till it coincides with the wire of the collimator; the amount by which it has to be moved giving an index of the error of collimation; that is to say, of the deviation of the optical axis of the telescope from perpendicularity to the line joining the pivots.
I have said enough to show that the making of an observation is a small matter as compared with those corrections which have to be made to it afterwards, before it is available for use. But I have only mentioned some of the reductions and corrections which have to be made. There are several more, and it is a just pride of Greenwich that her third ruler, Bradley, as has been already told in the notice of his life, discovered two of the most important.[201] The one, aberration, is due to the fact that light, though it moves so swiftly—186,000 miles per second—yet does not move with an infinitely greater velocity than that of the earth. The other, nutation, might be called a correction to precession, inasmuch as, moved by the moon's attraction, the earth's axis does not swing round smoothly, but with a slight nodding or staggering motion.
But when these observations of the places of a star have been made, and have been properly 'reduced,' even then we do not find an exact correspondence between two different determinations. Little differences still remain. Some of these are to be accounted for by changes in the actual crust of the earth, which, solid and stable as we think it, is yet always in motion. Professor Milne, our greatest authority on earth movements, says, 'The earth is so elastic that a comparatively small impetus will set it vibrating; why, even two hills tip together when there is a heavy load of moisture in the valley between them. And then, when the moisture evaporates in a hot sun, they tip away from each other.' So there is a perceptible rocking to and fro even of the huge stone piers of a transit circle, as seasons of rain and drought, heat and cold, follow each other. More than that, the earth is so sensitive to pressure that it was found, at the Oxford University Observatory, that there was a distinct swaying shown by a horizontal pendulum when the whole of a party of seventy-six undergraduates stood on one side of the instrument and close up to it, from the position it had when the party stood ninety feet away. More[202] wonderful still, a comparison of the star-places, obtained at a number of observatories, including Greenwich, has shown that the earth is continually changing her axis of rotation. And so the star-places determined at Greenwich have shown that the north pole of the earth, 2600 miles away, moves about in an irregular curve about thirty feet in radius.
Nothing is stable, nothing is immovable, nothing is constant. The astronomer even finds that his own presence near the instrument is sufficient to disturb it.
The great interest attaching to transit-circle work is this striving after ever greater and greater precision, with the result of bringing out fresh little discordances, which, at first sight, appear purely accidental, but which, under further scrutiny, show themselves to be subject to some law. Then comes the hunt for this new unknown law. Its discovery follows. It explains much, but when it is allowed for, though the observations now come much closer together, little deviations still remain, to form the subject of a fresh inquiry. Astronomy has well been called the exact science, and yet exactitude ever eludes its pursuer.
If it be asked, 'What is the use of this ever-increasing refinement of observation?' no better answer can be given than the words of Sir John Herschel in one of his Presidential addresses to the Royal Astronomical Society:—
'If we ask to what end magnificent establishments are maintained by States and sovereigns, furnished with masterpieces of art, and placed under the direction of men of first-rate talent and high-minded enthusiasm, sought out for those qualities among the foremost in the ranks of science,[203] if we demand, cui bono? for what good a Bradley has toiled, or a Maskelyne or a Piazzi has worn out his venerable age in watching?—the answer is, Not to settle mere speculative points in the doctrine of the universe; not to cater for the pride of man by refined inquiries into the remoter mysteries of nature; not to trace the path of our system through space, or its history through past and future eternities. These, indeed, are noble ends, and which I am far from any thought of depreciating; the mind swells in their contemplation, and attains in their pursuit an expansion and a hardihood which fit it for the boldest enterprise. But the direct practical utility of such labours is fully worthy of their speculative grandeur. The stars are the landmarks of the universe; and, amidst the endless and complicated fluctuations of our system, seem placed by its Creator as guides and records, not merely to elevate our minds by the contemplation of what is vast, but to teach us to direct our actions by reference to what is immutable in His works. It is, indeed, hardly possible to over-appreciate their value in this point of view. Every well-determined star, from the moment its place is registered, becomes to the astronomer, the geographer, the navigator, the surveyor, a point of departure which can never deceive or fail him, the same for ever and in all places; of a delicacy so extreme as to be a test for every instrument yet invented by man, yet equally adapted for the most ordinary purposes; as available for regulating a town clock as for conducting a navy to the Indies; as effective for mapping down the intricacies of a petty barony as for adjusting the boundaries of Transatlantic empires. When once its place has been thoroughly ascertained and carefully recorded, the brazen circle with which that useful work was done may moulder, the marble pillar may totter on its base, and the astronomer himself survive only in the gratitude of posterity; but the record remains, and transfuses all its own exactness into every determination which takes it for a groundwork, giving to inferior instruments—nay, even to temporary contrivances, and to the observations of a few weeks or days—all the precision attained originally at the cost of so much time, labour, and expense.'
But for these strictly utilitarian purposes a comparatively small number of stars would meet our every requisite. In the narrow sense of which Sir John Herschel is here speaking, we have no use for anything beyond the smallest of catalogues; and if the question before us is, 'Why are we continually extending our catalogues?' the following words of a more recent writer[4] on the subject will set forth the real explanation:—
'A word in conclusion, suggested by the history of star-catalogues. We have no difficulty in understanding that it is necessary to study the planets, and a reasonable number of the brighter stars, for the purpose of determining the figure of the earth, and the positions of points upon its surface; but the use for a catalogue of ten thousand stars, such as La Caille compiled, is not just so apparent. Nay, what did Ptolemy want with a thousand stars, or Tamerlane's grandson, born, reared, and destined to die amidst a horde of savages, however splendid in their trappings? There is not, and there never was, any real, practical use for the great volumes of star-catalogues that weigh down the shelves of our libraries. The navigator and explorer need never see them at all. Why, then, were these pages compiled? Why have astronomers, from Hipparchus's time to the present, spent their lives in the weary routine-work of observing the places of tiny points in the stellar depths? Does it not seem that there is something in the mind of man that impels him to seek after knowledge—truly—for its own sake? something heaven-born, heaven-nurtured, God-given ... that there is something in man common to him and his Creator, and therefore eternal ... in beautiful accord with the plain statement that "God made man in His own image?"'
CHAPTER VIII
THE ALTAZIMUTH DEPARTMENT
The determining of the places of the fixed stars which Flamsteed carried out so efficiently in his British Catalogue of Stars—the first 'Census of the Sky' made with the aid of a telescope—was but half of the work imposed upon him. The other half, equally necessary for the solution of the problem of the longitude at sea, was the 'Rectifying the Tables of the Motions of the Heavens.'
This second duty was not less necessary than the other, for, if we may again use the old simile of the clock-face, the fixed stars may be taken to represent the figures on the vast dial of the sky, whilst the moon, as it moves amongst them, corresponds to the moving hand of the timepiece. To know the places of the stars, then, without being able to predict the place of the moon, would be much like having a clock without its hands. But if not less necessary, it was certainly more difficult. The secret of the movements of the moon and planets had not then been grasped, and the only tables which had been calculated were based upon observations made before the days of telescopes.
It is one of the most fortunate and remarkable coincidences in the whole history of science, that at the very time that Greenwich Observatory was being called into existence, the greatest of all astronomers was working out his demonstration of the great fundamental law of the material universe—the law that every particle of matter attracts every other particle with a force which varies directly with the mass and inversely with the square of the distance.
Several other of the great minds of that time, in particular Dr. Hooke, the Gresham Professor of Astronomy, had seen that it was possible that some such law might supply the secret of planetary motion; but it is one thing to make a suggestion, and a very different matter indeed to be able to demonstrate it; and the latter was in Newton's power alone. He did much more than demonstrate it—he brought out a whole series of most important and far-reaching consequences. He showed that the ebb and flow of the tides was due to the attraction of both sun and moon, especially the latter, upon the waters of our oceans. He pointed out certain irregularities which must take place in the motion of our moon, due to the influence of the sun upon it. He showed, too, what was the cause of that swinging of the axis of the earth which gives rise to precession. He deduced the relative weights of the earth, the sun, and of Jupiter and Saturn, the planets with satellites. He proved also that comets, which had seemed hitherto to men as perfectly lawless wanderers, obeyed in their orbits the self-same law which governed the moon and planets. The whole vast system of celestial movements, which[207] had long seemed to men irregular and uncontrolled, now fell, every one of them, into its place, as but the necessary manifestations of one grand, simple order.
This great discovery gave a new and additional importance to the regular observation of the moon and planets. They were needed now, not only to assist in the practical work of navigation, but for the development of questions of pure science. Halley, the second Astronomer Royal, and Maskelyne, the fifth, devoted themselves chiefly to this department of work, to the partial neglect of the observation of the places of stars. Airy, the seventh, whilst making catalogue-work a part of the regular routine of the Observatory, developed the observation of the members of the solar system, and especially of the moon, in a most marked degree, and collected and completely reduced the vast mass of material which the industry of his predecessors had gathered. It is pre-eminently of the work of Airy that the memorable words quoted before of Professor Newcomb, the great American mathematician and astronomer, are applicable: 'that if this branch of astronomy were entirely lost, it could be reconstructed from the Greenwich observations alone.'
A most important step taken by Airy was the construction of an altazimuth. An altazimuth is practically a theodolite on a large scale. Its purpose is to determine, not the declination and right ascension of some celestial body, as is the case with the transit circle, but its altitude, i.e. its height above the horizon, and its azimuth, i.e. the angle measured on[208] the horizontal plane from the north point. The altazimuth, then, like the transit circle, consists of a telescope revolving on a horizontal axis, but, unlike the transit circle, both the telescope and the piers which carry its pivots can be rotated so as to point not merely due north and south, but in any direction whatsoever.
The observations with the altazimuth are rather more complicated than those with the transit circle.[209] Looking in the telescope, the observer sees a double set of spider threads or 'wires'; and when a star or other heavenly body enters the field, it will generally be observed to move obliquely across both sets of wires. The observer usually determines to make an observation either in altitude or azimuth. In the former case he presses the little contact button, which, as in the transit circle, is provided close to the eyepiece, as the star reaches each of the horizontal wires in succession. If in azimuth, it is the times of crossing the vertical wires that are in like manner telegraphed to the chronograph. The transit over, the appropriate circle is read; for the telescope itself is rigidly attached to a vertical wheel having a carefully engraved circle on its face and read by four microscopes, whilst the entire instrument carries another set of microscopes, pointing to a fixed horizontal circle, and upon which the azimuth can be read. A complete observation involves four such transits and sets of circle readings, two of altitude, and two of azimuth; for after one of altitude and one of azimuth the telescope is turned round, and a second observation is taken in each element.
The observation gives us the altitude and azimuth of the star. These particulars are of no direct value to us. But it is a mere matter of computation, though a long and laborious one, to convert these elements into right ascension and declination.
The usefulness of the altazimuth will be seen at once. It will be remembered that with the transit circle any particular object can only be observed as it crosses the meridian. If the weather should be[210] cloudy, or the observer late, the chance of observation is lost for four and twenty hours, and in the case of the moon, for which the altazimuth is specially used, it is on the meridian only in broad daylight during that part of the month which immediately precedes and follows new moon. At such times it is practically impossible to observe it with the transit circle; with the altazimuth it may be caught in the twilight before sunrise or after sunset; and at other times in the month, if lost on the meridian in the transit circle, the altazimuth still gives the observer a chance of catching it any time before it sets. But for this instrument, our observations of the moon would have been practically impossible over at least one-fourth of its orbit.
Airy's altazimuth was but a small instrument of three and three-quarter inches aperture, mounted in a high tower built on the site of Flamsteed's mural arc; and, after a life history of about half a century, has been succeeded by a far more powerful instrument. The 'New Altazimuth' has an aperture of eight inches, and is housed in a very solidly constructed building of striking appearance, the connection of the Observatory with navigation being suggested by a row of circular lights which strongly recall a ship's portholes. This building is at the southern end of the narrow passage, 'the wasp's waist,' which connects the older Observatory domain with the newer. It is the first building we come to in the south ground. The computations of the department are carried on in the south wing of the new Observatory.
It will be seen from the photograph that the[211] instrument is much larger, heavier, and less easy to move in azimuth than the old altazimuth. It is, therefore, not often moved in azimuth, but is set in some particular direction, not necessarily north and south, in which it is used practically as a transit circle.
There is quite another way of determining the place of the moon, which is sometimes available, and which offers one of the prettiest of observations to[212] the astronomer. As the moon travels across the sky, moving amongst the stars from west to east, it necessarily passes in front of some of them, and hides them from us for a time. Such a passage, or 'occultation,' offers two observations: the 'disappearance,' as the moon comes up to the star and covers it; the 'reappearance,' as it leaves it again, and so discloses it.
Except at the exact time of full moon, we do not see the entire face of our satellite; one edge or 'limb' is in darkness. As the moon therefore passes over the star, either the limb at which the star disappears, or that at which it reappears, is invisible to us. To watch an occultation at the bright limb is pretty; the moon, with its shining craters and black hollows, its mountain ranges in bright relief, like a model in frosted silver, slowly, surely, inevitably comes nearer and nearer to the little brilliant which it is going to eclipse. The movement is most regular, most smooth, yet not rapid. The observer glances at his clock, and marks the minute as the two heavenly bodies come closer and closer to each other. Then he counts the clock beats: 'five, six, seven,' it may be, as the star has been all but reached by the advancing moon. 'Eight,' it is still clear; ere the beat of the clock rings to the 'nine,' perhaps the little diamond point has been touched by the wide arch of the moon's limb, and has gone! Less easy to exactly time is a reappearance at the bright limb. In this case the observer must ascertain from the Nautical Almanac precisely where the star will reappear; then a little before the predicted time he takes his place at the
[215] telescope, watches intently the moon's circumference at the point indicated, and, listening for the clock-beats, counts the seconds as they fly. Suddenly, without warning, a pin-point of light flashes out at the edge of the moon, and at once draws away from it. The star has 'reappeared.'
Far more striking is a disappearance or reappearance at the 'dark limb.' In this case the limb of the moon is absolutely invisible, and it may be that no part of the moon is visible in the field of the telescope. In this case the observer sees a star shining brightly and alone in the middle of the field of his telescope. He takes the time from his faithful clock, counting beat after beat, when suddenly the star is gone! So sudden is the disappearance that the novice feels almost as astonished as if he had received a slap in the face, and not unfrequently he loses all count or recollection of the clock beats. The reappearance at the dark limb is quite as startling; with a bright star it is almost as if a shell had burst in his very face, and it would require no very great imagination to make him think that he had heard the explosion. One moment nothing was visible; now a great star is shining down serenely on the watcher. A little practice soon enables the observer to accustom himself to these effects, and an old hand finds no more difficulty in observing an occultation of any kind than in taking a transit.
Such an observation is useful for more purposes than one. If the position of the star occulted is known—and it can be determined at leisure afterwards—we necessarily know where the limb of the moon[216] was at the time of the observation. Then the time which the moon took to pass over the star enables us to calculate the diameter of our satellite; the different positions of the moon relative to the star, as seen from different observatories, enable us to calculate its distance.
But if the disappearance takes place at the bright limb, the reappearance usually takes place at the dark, and vice versâ; and the two observations are not quite comparable. There is one occasion, however, when both observations are made under similar circumstances, namely, at the full. And if the moon happens also to be totally eclipsed, the occultations of quite faint stars can be successfully observed, much fainter than can ordinarily be seen close up to the moon. Total eclipses of the moon, therefore, have recently come to be looked upon as important events for the astronomer, and observatories the world over usually co-operate in watching them. October 4, 1884, was the first occasion when such an organised observation was made; there have been several since, and on these nights every available telescope and observer at Greenwich is called into action.
It may be asked why these different modes of observing the moon are still kept up, year in and year out. 'Do we not know the moon's orbit sufficiently well, especially since the discovery of gravitation?' No; we do not. This simple and beautiful law—simple enough in itself, gives rise to the most amazing complexity of calculation. If the earth and moon were the only two bodies in the universe, the problem would be a simple one. But[217] the earth, sun, and moon are members of a triple system, each of which is always acting on both of the others. More, the planets, too, have an appreciable influence, and the net result is a problem so intricate that our very greatest mathematicians have not thoroughly worked it out. Our calculations of the moon's motions need, therefore, to be continually compared with observation, need even to be continually corrected by it.
There is a further reason for this continual observation, not only in the case of the sun, which is our great standard star, since from it we derive the right ascensions of the stars, and it is also our great timekeeper; not only in that of the moon, but also in the case of the planets. Their places as computed need continually to be compared with their places as observed, and the discordances, if any, inquired into. The great triumph which resulted to science from following this course—to pure science, since Uranus is too faint a planet to be any help to the sailor in navigation—is well known. The observed movements of Uranus proved not to be in accord with computation, and from the discordances between calculation and observation Adams and Leverrier were able to predicate the existence of a hitherto unseen planet beyond—
'To see it, as Columbus saw America from Spain. Its movements were felt by them trembling along the far-reaching line of their analysis, with a certainty hardly inferior to that of ocular demonstration.'[5]
The discovery of Neptune was not made at Greenwich, and Airy has been often and bitterly attacked because he did not start on the search for the predicted planet the moment Adams addressed his first communication to him, and so allowed the French astronomer to engross so much of the honour of the exploit. The controversy has been argued over and over again, and we may be content to leave it alone here. There is one point, however, which is hardly ever mentioned, which must have had much effect in determining Airy's conduct. In 1845, the year in which Adams sent his provisional elements of the unseen disturbing planet to Airy, the largest telescope available for the search at Greenwich was an equatorial of only six and three-quarter inches aperture, provided with small and insufficient circles for determining positions, and housed in a very small and inconvenient dome; whilst at Cambridge, within a mile or so of Adams' own college, was the 'Northumberland' equatorial, of nearly twelve inches aperture, under the charge of the University Professor of Astronomy, Professor Challis, and which was then much the largest, best mounted and housed equatorial in the entire country. The 'Northumberland' had been begun from Airy's designs and under his own superintendence, when he was Professor of Astronomy at Cambridge. Naturally, then, knowing how much superior the Cambridge telescope was to any which he had under his care, he thought the search should be made with it. He had no reason to believe that his own instrument was competent for the work.
On the other hand, it is hard for the ordinary
[221]man to understand how it was that Adams not only left unnoticed and unanswered for three-quarters of a year, an inquiry of Airy's with respect to his calculations, but also never took the trouble to visit Challis, whom he knew well, and who was so near at hand, to stir him up to the search. But, in truth, the whole interest of the matter for Adams rested in the mathematical problem. The irregularities in the motion of Uranus were interesting to him simply for the splendid opportunity which they gave him for their analysis. A purely imaginary case would have served his purpose nearly as well. The actuality of the planet which he predicted was of very little moment; the éclat and popular reputation of the discovery was less than nothing; the problem itself and the mental exercise in its solution, were what he prized.
But it was not creditable to the nation that the Royal Observatory should have been so ill-provided with powerful telescopes; and a few years later Airy obtained the sanction of the Government for the erection of an equatorial larger than the 'Northumberland,' but on the same general plan and in a much more ample dome. This was for thirty-four years the 'Great' or 'South-East' equatorial, and the mounting still remains and bears the old name, though the original telescope has been removed elsewhere. The object-glass had an aperture of twelve and three-quarter inches and a focal length of eighteen feet, and was made by Merz of Munich, the engineering work by Ransomes and Sims of Ipswich, and the graduations and general optical work by Simms, now[222] of Charlton, Kent. The mounting was so massive and stable that the present Astronomer Royal has found it quite practicable and safe to place upon it a telescope (with its counterpoises) of many times the weight, one made by Sir Howard Grubb, of Dublin, of twenty-eight inches aperture and twenty-eight feet focal length, the largest refractor in the British Empire, though surpassed by several American and Continental instruments.
The stability of the mounting was intended to render the telescope suitable for a special work. This was the observation of 'minor planets.' On the first day of the present century the first of these little bodies was discovered by Piazzi at Palermo. Three more were discovered at no great interval afterwards, and then there was an interval of thirty-eight years without any addition to their number. But from December 8, 1845, up to the present time, the work of picking up fresh individuals of these 'pocket planets' has gone on without interruption, until now more than 400 are known. Most of these are of no interest to us, but a few come sufficiently near to the earth for their distance to be very accurately determined; and when the distance of one member of the solar system is determined, those of all the others can be calculated from the relations which the law of gravitation reveals to us. It is a matter of importance, therefore, to continue the work of discovery, since we may at any time come across an interesting or useful member of the family; and that we may be able to distinguish between minor planets already discovered and new ones, their orbits must[223] be determined as they are discovered, and some sort of watch kept on their movements.
A striking example of the scientific prizes which we may light upon in the process of the rather dreary and most laborious work which the minor planets cause, has been recently supplied by the discovery of Eros. On August 13, 1898, Herr Witt, of the Urania Observatory, Berlin, discovered a very small planet that was moving much faster in the sky than is common with these small bodies. The great majority are very much farther from the sun than the planet Mars, many of them twice as far, and hence, since the time of a planet's revolution round the sun increases, in accordance with Kepler's law, more rapidly than does its distance, it follows that they move much more slowly than Mars. But this new object was moving at almost the same speed as Mars; it must, therefore, be most unusually near to us. Further observations soon proved that this was the case, and Eros, as the little stranger has been called, comes nearer to us than any other body of which we are aware except the moon. Venus when in transit is 241/2 millions of miles from us, Mars at its nearest is 341/2 millions, Eros at its nearest approach is but little over 13 millions.
The use of such a body to us is, of course, quite apart from any purpose of navigation, except very indirectly. But it promises to be of the greatest value in the solution of a question in which astronomers must always feel an interest, the determination of the distance of the earth from the sun. We know the relative distances of the different planets, and,[224] consequently if we could determine the absolute distance of any one, we should know the distances of all. As it is practically impossible to measure our distance from the sun directly, several attempts have been made to determine the distances of Venus, Mars, or such of the minor planets as come the nearest to us. Three of these in particular, the little planets Iris, Victoria, and Sappho, have given us the most accurate determinations of the sun's distance (92,874,000 miles) which we have yet obtained; but Eros at its nearest approach will be six times as near to us as either of the three mentioned above, and therefore should give us a value with only one-sixth of the uncertainty attaching to that just mentioned.
The discovery of minor planets has lain outside the scope of Greenwich work, but their observation has formed an integral part of it. The general public is apt to lay stress rather on the first than on the second, and to think it rather a reproach to Greenwich that it has taken no part in such explorations. Experience has, however, shown that they may be safely left to amateur activity, whilst the monotonous drudgery of the observation of minor planets can only be properly carried out in a permanent institution.
The observation of these minute bodies with the transit circle and altazimuth is attended with some difficulties; but precise observations of various objects may be made with an equatorial; indeed, comets are usually observed by its means.
The most ordinary way of observing a comet with an equatorial is as follows: Two bars are placed in[225] the eye-piece of the telescope, at right angles to each other, and at an angle of forty-five degrees to the direction of the apparent daily motion of the stars. The telescope is turned to the neighbourhood of the comet, and moved about until it is detected. The telescope is then put a little in front of the comet, and very firmly fixed. The observer soon sees the comet entering his field, and by pressing the contact button he telegraphs to the chronograph the time when the comet is exactly bisected by each of the bars successively. He then waits until a bright star, or it may be two or three, have entered the telescope and been observed in like manner. The telescope is then unclamped, and moved forward until it is again ahead of the comet, and the observations are repeated; and this is done as often as is thought desirable. The places of the stars have, of course, to be found out from catalogues, or have to be observed with the transit circle, but when they are known the position of the comet or minor planet can easily be inferred.
Next to the glory of having been the means of bringing about the publication of Newton's Principia, the greatest achievement of Halley, the second Astronomer Royal, was that he was the first to predict the return of a comet. Newton had shown that comets were no lawless wanderers, but were as obedient to gravitation as were the planets themselves, and he also showed how the orbit of a comet could be determined from observations on three different dates. Following these principles, Halley computed the orbits of no fewer than twenty-four[226] comets, and found that three of them, visible at intervals of about seventy-five years, pursued practically the same path. He concluded, therefore, that these were really different appearances of the same object, and, searching old records, he found reason to believe that it had been observed frequently earlier still. It seems, in fact, to have been the comet which is recorded to have been seen in 1066 in England at the time of the Norman invasion; in A.D. 66, shortly before the commencement of that war which ended in the destruction of Jerusalem by Titus; and earlier still, so far back as B.C. 12. Halley, however, experienced a difficulty in his investigation. The period of the comet's revolution was not always the same. This, he concluded, must be due to the attraction of the planets near which the comet might chance to travel. In the summer of 1681 it had passed very close to Jupiter, for instance, and in consequence he expected that instead of returning in August 1757, seventy-five years after its last appearance, it would not return until the end of 1758 or the beginning of 1759. It has returned twice since Halley's day, a triumphant verification of the law of gravitation; and we are looking for it now for a third return some ten years hence, in 1910.
Halley's comet, therefore, is an integral member of our solar system, as much so as the earth or Neptune, though it is utterly unlike them in appearance and constitution, and though its path is so utterly unlike theirs that it approaches the sun nearer than our earth, and recedes farther than Neptune. But there are other comets, which are[227] not permanent members of our system, but only passing visitors. From the unfathomed depths of space they come, to those depths they go. They obey the law of gravitation so far as our sight can follow them, but what happens to them beyond? Do they come under some other law, or, perchance, in outermost space is there still a region reserved to primeval Chaos, the 'Anarch old,' where no law at all prevails? Gravitation is the bond of the solar system; is it also the bond of the Universe?
CHAPTER IX
THE MAGNETIC AND METEOROLOGICAL DEPARTMENTS
Passing out of the south door of the new altazimuth building, we come to a white cruciform erection, constructed entirely of wood. This is the Magnet House or Magnetic Observatory, the home of a double Department, the Magnetic and Meteorological.
This department does not, indeed, lie within the original purpose of the Observatory as that was defined in the warrant given to Flamsteed, and yet is so intimately connected with it, through its bearing on navigation, that there can be no question as to its suitability at Greenwich. Indeed, its creation is a striking example of the thorough grasp which Airy had upon the essential principles which should govern the great national observatory of an essentially naval race, and of the keen insight with which he watched the new development of science. The Magnetic Observatory, therefore, the purpose of which was to deal with the observation of the changes in the force and direction of the earth's magnetism—an inquiry which the greater delicacy of modern compasses, and, in more recent times, the use of iron[229] instead of wood in the construction of ships has rendered imperative—was suggested by Airy on the first possible occasion after he entered on his office, and was sanctioned in 1837. The Meteorological Department has a double bearing on the purpose of the Observatory. On the one side, a knowledge of the temperature and pressure of the atmosphere is, as we have already seen, necessary in order to correct astronomical observations for the effect of refraction. On the other hand, meteorology proper, the study of the movements of the atmosphere, the elucidation of the laws which regulate those movements, leading to accurate forecasts of storms, are of the very first necessity for the safety of our shipping. It is true that weather forecasts are not issued from Greenwich Observatory, any more than the Nautical Almanac is now issued from it; but just as the Observatory furnishes the astronomical data upon which the Almanac is based, so also it takes its part in furnishing observations to be used by the Meteorological Office at Westminster for its daily predictions.
Those predictions are often made the subject of much cheap ridicule; but, however far short they may fall of the exact and accurate predictions which we would like to have, yet they mark an enormous advance upon the weather-lore of our immediate forefathers.
says the proverb, and the saying is not without a shrewd amount of truth. For perhaps nowhere can we find a more striking combination of imperfect[230] observation and inconsequent deduction than in the saws which form the stock-in-trade of the ordinary would-be weather prophet. How common it is to find men full of the conviction that the weather must change at the co-called 'changes of the moon,' forgetful that
They will say, truly enough, no doubt, that they have known the weather to change at 'new' or 'full,' as the case may be, and they argue that it, therefore, must always do so. But, in fact, they have only noted a few chance coincidences, and have let the great number of discordances pass by unnoticed.
But observations of this kind seem scientific and respectable compared with those numerous weather proverbs which are based upon the mere jingle of a rhyme, as
a proverb which is deftly inverted in some districts by making 'oak' rhyme to 'choke.'
Others, again, are based upon a mere childish fancy, as, for example, when the young moon 'lying on her back' is supposed to bode a spell of dry weather, because it looks like a cup, and so might be thought of as able to hold the water.
During the present reign, however, a very different method of weather study has come into action, and[231] the foundations of a true weather wisdom have been laid. These have been based, not on fancied analogies or old wives' rhymes, or a few forechosen coincidences, but upon observations carried on for long periods of time and over wide areas of country, and discussed in their entirety without selection and bias. Above all, mathematical analysis has been applied to the motions of the air, and ideas, ever gaining in precision and exactness, have been formulated of the general circulation of the atmosphere.
As compared with its sister science, astronomy, meteorology appears to be still in a very undeveloped state. There is such a difference between the power of the astronomer to foretell the precise position of sun, moon, and planets for years, even for centuries, beforehand, and the failure of the meteorologist to predict the weather for a single season ahead, that the impression has been widely spread that there is yet no true meteorological science at all. It is forgotten that astronomy offered us, in the movements of the heavenly bodies, the very simplest and easiest problem of related motion. Yet for how many thousands of years did men watch the planets, and speculate concerning their motions, before the labours of Tycho, Kepler, and Newton culminated in the revelation of their meaning? For countless generations it was supposed that their movements regulated the lives, characters, and private fortunes of individual men; just as quite recently it was fancied that a new moon falling on a Saturday, or two full moons coming within the same calendar month, brought bad weather!
It is still impossible to foresee the course of weather change for long ahead; but the difference between the modern navigator, surely and confidently making a 'bee-line' across thousands of miles of ocean to his destination, and the timid sailor of old, creeping from point to point of land, is hardly greater than the contrast between the same two men, the one watching his barometer, the other trusting in the old wives' rhymes which afforded him his only indication as to coming storms.
It is still impossible to foresee the weather change for long ahead, but in our own and in many other countries, especially the United States, it has been found possible to predict the weather of the coming four-and-twenty hours with very considerable exactness, and often to forecast the coming of a great storm several days ahead. This is the chief purpose of the two great observatories of the storm-swept Indian and Chinese seas, Hong Kong and Mauritius; and the value of the work which they have done in preventing the loss of ships, and the consequent loss of lives and property, has been beyond all estimate.
The Royal Observatory, Greenwich, is a meteorological as well as an astronomical observatory, but, as remarked above, it does not itself issue any weather forecasts. Just as the Greenwich observations of the places of the moon and stars are sent to the Nautical Almanac Office, for use in the preparation of that ephemeris; just as the Greenwich determinations of time are used for the issue of signals to the Post Office, whence they are distributed over the kingdom, so the Greenwich observations of weather are sent to the[233] Meteorological Office, there to be combined with similar records from every part of the British Isles, to form the basis of the daily forecasts which the latter office publishes. To each of these three offices, therefore, the Royal Observatory, Greenwich, stands in the relation of purveyor. It supplies them with the original observations more or less in reduced and corrected form, without which they could not carry on most important portions of their work.
Let it be noted how closely these three several departments, the Nautical Almanac Office, the Time Department, and the Meteorological Office, are related to practical navigation. Whatever questions of pure science—of knowledge, that is, apart from its useful applications—may arise out of the following up of these several inquiries, yet the first thought, the first principle of each, is to render navigation more sure and more safe.
The first of all meteorological instruments is the barometer, which, under its two chief forms of mercurial and aneroid, is simply a means of measuring the pressure exerted by the atmosphere.
There are two important corrections to which its readings are subject. The first is for the height of the station above the level of the sea; the second is for the effect of temperature upon the mercury in the barometer itself, lengthening the column. To overcome these, the height of the standard barometer at Greenwich above sea-level has been most carefully ascertained, and the heights relative to it of the other barometers of the Observatory, particularly those in rooms occupied by fundamental telescopes, have also[234] been determined, whilst the self-recording barometer is mounted in a basement, where it is almost completely protected from changes of temperature.
Next in importance to the barometer as a meteorological instrument comes the thermometer. The great difficulty in the Observatory use of the thermometer is to secure a perfectly unexceptionable exposure, so that the thermometer may be in free and perfect contact with the air, and yet completely sheltered from any direct ray from the sun. This is secured in the great thermometer shed at Greenwich by a double series of 'louvre' boards, on the east, south, and west sides of the shed, the north side being open. The shed itself is made a very roomy one, in order to give access to a greater body of air.
A most important use of the thermometer is in the measurement of the amount of moisture in the air. To obtain this, a pair of thermometers are mounted close together, the bulb of one being covered by damp muslin, and the other being freely exposed. If the air is completely saturated with moisture, no evaporation can take place from the damp muslin, and consequently the two thermometers will read the same. But if the air be comparatively dry, more or less evaporation will take place from the wet bulb, and its temperature will sink to that at which the air would be fully saturated with the moisture which it already contained. For the higher the temperature, the greater is its power of containing moisture. The difference of the reading of the two thermometers is, therefore, an index of humidity. The greater the difference, the greater the power of absorbing moisture,
[237]or, in other words, the dryness of the air. The great shed already alluded to is devoted to these companion thermometers.
Very closely connected with atmospheric pressure, as shown us by the barometer, is the study of the direction of winds. If we take a map of the British Isles and the neighbouring countries, and put down upon them the barometer readings from a great number of observing stations, and then join together the different places which show the same barometric pressure, we shall find that these lines of equal pressure—technically called 'isobars'—are apt to run much nearer together in some places than in others. Clearly, where the isobars are close together it means that in a very short distance of country we have a great difference of atmospheric pressure. In this case we are likely to get a very strong wind blowing from the region of high pressure to the region of low pressure, in order to restore the balance.
If, further, we had information from these various observing stations of the direction in which the wind was blowing, we should soon perceive other relationships. For instance, if we found that the barometer read about the same in a line across the country from east to west, but that it was higher in the north of the islands than in the south, we should then have a general set of winds from the east, and a similar relation would hold good if the barometer were highest in some other quarter; that is, the prevailing wind will come from a quarter at right angles to the region of highest barometer, or, as it is expressed in what is known as 'Buys Ballot's law,'[238] 'stand with your back to the wind, and the barometer will be lower on your left hand than on your right.' This law holds good for the northern hemisphere generally, except near to the equator; in the southern hemisphere the right hand is the side of low barometer.
The instruments for wind observation are of two classes: vanes to show its direction, and anemometers to show its speed and its pressure. These may be regarded as two different modes in which the strength of the wind manifests itself. Pressure anemometers are usually of two forms: one in which a heavy plate is allowed to swing by its upper edge in a position fronting the wind, the amount of its deviation from the vertical being measured; and the other in which the plate is supported by springs, the degree of compression of the springs being the quantity registered in that case. Of the speed anemometers, the best known form is the 'Robinson,' in which four hemispherical cups are carried at the extremities of a couple of cross bars.
For the mounting of these wind instruments the old original Observatory, known as the Octagon Room, has proved an excellent site, with its flat roof surmounted by two turrets in the north-east and north-west corners, and raised some two hundred feet above high-water mark.
The two chief remaining instruments are those for measuring the amount of rainfall and of full sunshine. The rain gauge consists essentially of a funnel to collect the rain, and a graduated glass to measure it. The sunshine recorder usually consists of a large glass globe arranged to throw an image
[241] of the sun on a piece of specially prepared paper. This image, as the sun moves in the sky, moves along the paper, charring it as it moves, and at the end of the day it is easy to see, from the broken, burnt trace, at what hours the sun was shining clear, and when it was hidden by cloud.
An amusing difficulty was encountered in an attempt to set on foot another inquiry. The Superintendent of the Meteorological Department at the time wished to have a measure of the rate at which evaporation took place, and therefore exposed carefully measured quantities of water in the open air in a shallow vessel. For a few days the record seemed quite satisfactory. Then the evaporation showed a sudden increase, and developed in the most erratic and inexplicable manner, until it was found that some sparrows had come to the conclusion that the saucer full of water was a kindly provision for their morning 'tub,' and had made use of it accordingly.
A large proportion of the meteorological instruments at Greenwich and other first-class observatories are arranged to be self-recording. It was early felt that it was necessary that the records of the barometer and thermometer should be as nearly as possible continuous; and at one time, within the memory of members of the staff still living, it was the duty of the observer to read a certain set of instruments at regular two-hour intervals during the whole of the day and night—a work probably the most monotonous, trying, and distasteful of any that the Observatory had to show.
The two-hour record was no doubt practically[242] equivalent to a continuous one, but it entailed a heavy amount of labour. Automatic registers were, therefore, introduced whenever they were available. The earliest of these were mechanical, and several still make their records in this manner.
On the roof of the Octagon Room we find, beside the two turrets already referred to, a small wooden cabin built on a platform several feet above the roof level. This cabin and the north-western turret contain the wind-registering instruments. Opening the turret door, we find ourselves in a tiny room which is nearly filled by a small table. Upon this table lies a graduated sheet of paper in a metal frame, and as we look at it, we see that a clock set up close to the table is slowly drawing the paper across it. Three little pencils rest lightly on the face of the paper at different points. One of these, and usually the most restless, is connected with a spindle which comes down into the turret from the roof, and which is, in fact, the spindle of the wind vane. The gearing is so contrived that the motion on a pivot of the vane is turned into motion in a straight line at right angles to the direction in which the paper is drawn by the clock. A second pencil is connected with the wind-pressure anemometer. The third pencil indicates the amount of rain that has fallen since the last setting, the pencil being moved by a float in the receiver of the rain gauge.
An objection to all the mechanical methods of continuous registration is that, however carefully the gearing between the instrument itself and the pencil is contrived, however lightly the pencil moves over[243] the paper, yet some friction enters in and affects the record: this is of no great moment in wind registration, when we are dealing with so powerful an agent as the wind, but it becomes a serious matter when the[244] barometer is considered, since its variations require to be registered with the greatest minuteness. When photography, therefore, was invented, meteorologists were very prompt to take advantage of this new ally. A beam of light passing over the head of the column of mercury in a thermometer or barometer could easily be made to fall upon a drum revolving once in the twenty-four hours, and covered with a sheet of photographic paper. In this case, when the sensitive paper is developed, we find its upper half blackened, the lower edge of the blackened part showing an irregular curve according as the mercury in the thermometer or barometer rose or fell, and admitted less or more light through the space above it.
Here we have a very perfect means of registration: the passage of the light exercises no friction or check on the free motion of the mercury in the tube, or on the turning of the cylinder covered by the sensitive paper, whilst it is easy to obtain a time scale on the register by cutting off the light for an instant—say at each hour. In this way the wet and dry bulb thermometers in the great shed make their registers.
The supply of material to the Meteorological Office is not the only use of the Greenwich meteorological observations. Two elements of meteorology, the temperature and the pressure of the atmosphere, have the very directest bearing upon astronomical work. And this in two ways. An instrument is sensible to heat and cold, and undergoes changes of form, size, or scale, which, however absolutely minute, yet become, with the increased delicacy of modern work, not merely appreciable, but important. So too[245] with the density of the atmosphere: the light from a distant star, entering our atmosphere, suffers refraction; and being thus bent out of its path, the star appears higher in the heavens than it really is. The amount of this bending varies with the density of the layers of air through which the light has to pass. The two great meteorological instruments, the thermometer and barometer, are therefore astronomical instruments as well.
In the arrangements at Greenwich the Magnetic Department is closely connected with the Meteorological, and it is because the two departments have been associated together that the building devoted to both is constructed of wood, not brick, since ordinary bricks are made of clay which is apt to be more or less ferruginous. Copper nails have alone been employed in the construction of the buildings. The fire-grates, coal-scuttles, and fire-irons are all of the same metal.
The growth of the Observatory has, however, made it necessary to set up some of the new telescopes, into the mounting of which much iron enters, very close to the magnetic building. The present Astronomer-Royal has therefore erected a Magnetic Pavilion right out in the park at an ample distance from these disturbing causes.
The double department is, therefore, the most widely scattered in the whole Observatory. It is located for computing purposes in the west wing of the New Observatory; many of its magnetic instruments are in the old Magnet House, others are across the park in the new Magnetic Pavilion; the[246] anemometers are on the roof of the Octagon Room, Flamsteed's original observatory, and the self-registering thermometers are in the south ground between the old Magnet House and the New Observatory.
The object of the Magnetic Observatory is to study the movements of the magnetic needle. The quaintest answer that I ever received in an examination was in reply to the question, 'What is meant by magnetic inclination and declination?' The examinee replied:
'To make a magnet, you take a needle, and rub it on a lodestone. If it refuses or declines to become a magnet, that is magnetic declination; if it is easily made a magnet, or is inclined to become one, that is magnetic inclination.'
One greatly regretted that it was necessary to mark the reply according to its ignorance, and not, as one would have wished, in proportion to its ingenuity. Magnetic declination, however, as everybody knows, measures the deviation of the 'needle' from the true geographical north and south direction; the inclination or dip is the angle which a 'needle' makes with the horizon.
At one time the only method of watching the movements of the magnetic needles was by direct observation, just precisely as it was wont to be in the case of the barometer and thermometer. But the same agent that has been called in to help in their case has enabled the magnets also to give us a direct and continuous record of their movements. In principle the arrangement is as follows: A small light mirror is attached to the magnetic needle, and a beam of light is arranged to fall upon the mirror, and is reflected away from it to a drum covered with sensitive paper. If, then, the needle is perfectly at rest, a spot of light falls on the drum and blackens the paper at one particular point. The drum is made to revolve by clockwork once in twenty-four hours, and the black dot is therefore lengthened out into a straight line encircling the drum. If, however, the needle moves, then the spot of light travels up or down, as the case may be.
Now, if we look at one of these sheets of photographic paper after it has been taken from the drum, we shall see that the north pole of the magnet has moved a little, a very little, towards the west in the early part of the day, say from sunrise to 2 p.m., and has swung backwards from that hour till about 10 p.m., remaining fairly quiet during the night. The extent of this daily swing is but small, but it is greater in summer than in winter, and it varies also from year to year.
Besides this daily swing, there occasionally happen what are called 'magnetic storms;' great convulsive twitchings of the needle, as if some unseen operator[249] were endeavouring, whilst in a state of intense excitement, to telegraph a message of vast importance, so rapid and so sharp are the movements of the needle to and fro. These great storms are felt, so far as we know, simultaneously over the whole earth, and the more characteristic begin with a single sharp twitch of the needle towards the east.
Besides the movements of the magnetic needle, the intensity of the currents of electricity which are always passing through the crust of the earth are also determined at Greenwich; but this work has been rendered practically useless for the last few years by the construction of the electric railway from Stockwell to the City. Since it was opened, the photographic register of earth currents has shown a broad blurring from the moment of the starting of the first train in the morning to the stopping of the last train at night. As an indication of the delicacy of modern instruments, it may be mentioned that distinct indications of the current from this railway have been detected as far off as North Walsham, in Norfolk, a distance of more than a hundred miles. A further illustration of the delicacy of the magnetic needles was afforded shortly after the opening of the railway referred to. On one occasion the then Superintendent of the Magnetic Department visited the Generating Station at Stockwell, and on his return it was noticed day after day that the traces from the magnets showed a curious deflection from 9 a.m. to 3 p.m., the hours of his attendance. This gave rise to some speculation, as it did not seem possible that the gentleman could himself have become magnetized.[250] Eventually, the happy accident of a fine day solved the mystery. That morning the Superintendent left his umbrella at home, and the magnets were undisturbed. The secret was out. The umbrella had become a permanent magnet, and its presence in the lobby of the magnetic house had been sufficient to influence the needles.
CHAPTER X
THE HELIOGRAPHIC DEPARTMENT
So far the development of the Observatory had been along the central line of assistance to navigation. But the Magnetic Department led on to one which had but a very secondary connection with it.
A greatly enhanced interest was given to the observations of earth magnetism, when it was found that the intensity and frequency of its disturbances were in close accord with changes that were in progress many millions of miles away. That the surface of the sun was occasionally diversified by the presence of dark spots, had been known almost from the first invention of the telescope; but it was not until the middle of the present century that any connection was established between these solar changes and the changes which took place in the magnetism of the earth. Then two observers, the one interesting himself entirely with the spots on the sun, the other as wholly devoted to the study of the movements of the magnetic needle, independently found that the particular phenomenon which each was watching was one which varied in a more or less regular cycle. And further, when the cycles were[252] compared, they proved to be the same. Whatever the secret of the connection, it is now beyond dispute that as the spots on the sun become more and more numerous, so the daily swing of the magnetic needle becomes stronger; and, on the other hand, as the spots diminish, so the magnetic needle moves more and more feebly.
This discovery has given a greatly increased significance to the study of the earth's magnetism. The daily swing, the occasional 'storms,' are seen to be something more than matters of merely local interest; they have the closest connection with changes going on in the vast universe beyond; they have an astronomical importance.
And it was soon felt to be necessary to supplement the Magnetic Observatory at Greenwich by one devoted to the direct study of the solar surface; and here again that invaluable servant of modern science, photography, was ready to lend its help. Just as, by the means of photography, the magnets recorded their own movements, so even more directly the sun himself makes register of his changes by the same agency, and gives us at once his portrait and his autograph.
This new department was again due to Airy, and in 1873 the 'Kew' photo-heliograph, which had been designed by De la Rue for this work, was installed at Greenwich.
In order to photograph so bright a body as the sun, it is not in the least necessary to have a very large telescope. The one in common use at Greenwich from 1875 to 1897, is only four inches in aperture
[255] and even that is usually diminished by a cap to three inches, and its focal length is but five feet. This is not very much larger than what is commonly called a 'student's telescope,' but it is amply sufficient for its work.
This 'Dallmeyer' telescope, so called from the name of its maker, is one of five identical instruments which were made for use in the observation of the transit of Venus of 1874, and which, since they are designed for photographing the sun, are called 'photo-heliographs.'
The image of the sun in the principal focus of this telescope is about six-tenths of an inch in diameter; but a magnifying lens is used, so that the photograph actually obtained is about eight inches. Even with this great enlargement, the light of the sun is so intense that with the slowest photographic plates that are made the exposure has to be for only a very small fraction of a second. This is managed by arranging a very narrow slit in a strip of brass. The strip is made to run in a groove across the principal focus. Before the exposure, it is fastened up so as to cut off all light from entering the camera part of the telescope. When all is ready, it is released and drawn down very rapidly by a powerful spring, and the slit, flying across the image of the sun, gives exposure to the plate for a very minute fraction of a second—in midsummer for less than a thousandth of a second.
Two of these photographs are taken every fine day at Greenwich; occasionally more, if anything specially interesting appears to be going on. But in our cloudy climate at least one day in three gives no[256] good opportunity for taking photographs of the sun, and in the winter time long weeks may pass without a chance. The present Astronomer-Royal, Mr. Christie, has therefore arranged that photographs with precisely similar instruments should be taken in India and in the Mauritius, and these are sent over to Greenwich as they are required, to fill up the gaps in the Greenwich series. We have therefore at Greenwich, from one source or another, practically a daily record of the state of the sun's surface.
More recently the 'Dallmeyer' photo-heliograph, though still retained for occasional use, has been superseded generally by the 'Thompson'; a photographic refractor of nine inches aperture, and nearly nine feet focal length, presented to the Observatory by Sir Henry Thompson. The image of the sun obtained after enlargement in the telescope, with this instrument, is seven and a half inches in diameter. The 'Thompson' is mounted below the great twenty-six-inch photographic refractor,—also presented to the Observatory by Sir Henry Thompson,—in the dome which crowns the centre of the New Observatory.
A photograph of the sun taken, it has next to be measured, the four following particulars being determined for each spot: First, its distance from the centre of the image of the sun; next, the angle between it and the north point; thirdly, the size of the spot; and fourthly, the size of the umbra of the spot, that is to say, of its dark central portion. The size or area of the spot is measured by placing a thin piece of glass, on which a number of cross-lines have been ruled one-hundredth of an inch apart, in contact[257] with the photograph. These cross-lines make up a number of small squares, each the ten-thousandth (1/10000 in.) part of a square inch in area. When the photograph and the little engraved glass plate are nearly in contact, the photograph is examined with a magnifying glass, and the number of little squares covered by a given spot are counted. It will give some idea of the vast scale of the sun when it is stated that a tiny spot, so small that it only just covers one of these little squares, and which is only one-millionth of the visible hemisphere of the sun in area, yet covers in actual extent considerably more than one million of square miles.
The dark spots are not the only objects on the sun's surface. Here and there, and especially near the edge of the sun, are bright marks, generally in long branching lines, so bright as to appear bright even against the dazzling background of the sun itself. These are called 'faculæ,' and they, like the spots, have their times of great abundance and of scarcity, changing on the whole at the same time as the spots.
After the solar photographs have been measured, the measures must be 'reduced,' and the positions of the spots as expressed in longitude and latitude on the sun computed. There is no difficulty in doing this, for the position of the sun's equator and poles have long been known approximately, the sun revolving on its axis in a little more than twenty-five days, and carrying of course the spots and faculæ round with him.
There are few studies in astronomy more engrossing than the watch on the growth and changes of the[258] solar spots. Their strange shapes, their rapid movements, and striking alterations afford an unfailing interest. For example, the amazing spectacle is continually being afforded of a spot, some two, three, or four hundred millions of square miles in area, moving over the solar surface at a speed of three hundred miles an hour, whilst other spots in the same group are remaining stationary. But a higher interest attaches to the behaviour of the sun as a whole than to the changes of any particular single spot; and the curious fact has been brought to light, that not only do the spots increase and diminish in a regular cycle of about eleven years in length, but they also affect different regions of the sun at different points of the cycle. At the time when spots are most numerous and largest, they are found occupying two broad belts, the one with its centre about 15° north of the equator, the other about as far south, the equator itself being very nearly free from them. But as the spots begin to diminish, so they appear continually in lower and lower latitudes, until instead of having two zones of spots there is only one, and this one lies along the equator. By this time the spots have become both few and small. The next stage is that a very few small spots are seen from time to time in one hemisphere or the other at a great distance from the equator, much farther than any were seen at the time of greatest activity. There are then for a little time three sun-spot belts, but the equatorial one soon dies out. The two belts in high latitude, on the other hand, continually increase; but as they increase, so do they move downwards in latitude, until at length
[261]they are again found in about latitude 15° north or south, when the spots have attained their greatest development.
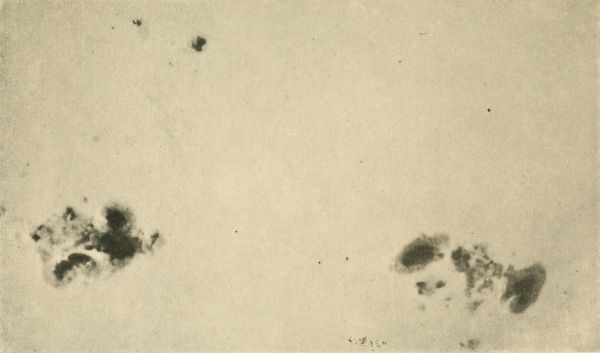
PHOTOGRAPH OF A GROUP OF SUN-SPOTS.
(From a photograph taken at the Royal Observatory, Greenwich, April, 1882, 20 d. 10 h. 6 m.)
The clearest connection between the magnetic movements and the sun-spot changes is seen when we take the mean values of either for considerable periods of time, as, for instance, year by year. But occasionally we have much more special instances of this connection. Some three or four times within the last twenty years an enormous spot has broken out on the sun, a spot so vast that worlds as great as our own could lie in it like peas in a breakfast saucer, and in each case there has been an immediate and a threefold answer from the earth. One of the most remarkable of these occurred in November, 1882. A great spot was then seen covering an area of more than three thousand millions of square miles. The weather in London happened to be somewhat foggy, and the sun loomed, a dull red ball, through the haze, a ball it was perfectly easy to look at without specially shading the eyes. So large a spot under such circumstances was quite visible to the naked eye, and it caught the attention of a great number of people, many of whom knew nothing about the existence of spots on the sun.
This great disturbance, evidently something of the nature of a storm in the solar atmosphere, stretched over one hundred thousand miles on the surface of the sun. The disturbance extended farther still, even to nearly one hundred millions of miles. For simultaneously with the appearance of the spot the magnetic needles at Greenwich began[262] to suffer from a strange excitement, an excitement which grew from day to day until it had passed half-way across the sun's disc. As the twitchings of the magnetic needle increased in frequency and violence, other symptoms were noticed throughout the length of the British Isles. Telegraphic communication was greatly interfered with. The telegraph lines had other messages to carry more urgent than those of men. The needles in the telegraph instruments twitched to and fro. The signal bells on many of the railway lines were rung, and some of the operators received shocks from their instruments. Lastly, on November 17, a superb aurora was witnessed, the culminating feature of which was the appearance, at about six o'clock in the evening, of a mysterious beam of greenish light, in shape something like a cigar, and many degrees in length, which rose in the east and crossed the sky at a pace much quicker than but nearly as even as that of sun, moon, or stars, till it set in the west two minutes after its rising.
So far we have been dealing only with effects. Their causes still rest hidden from us. There is clearly a connection between the solar activity as shown by the spots and the agitation of the magnetic needles. But many great spots find no answer in any magnetic vibration, and not a few considerable magnetic storms occur when we can detect no great solar changes to correspond.
Thus even in the simplest case before us we have still very much to explain. Two far more difficult problems are still offered us for solution. What is[263] the cause of these mysterious solar spots? and have they any traceable connection with the fitful vagaries of earthly weather? It was early suggested that probably the first problem might find an answer in the ever-varying combinations and configurations of the various planets, and that the sun-spots in their turn might hold the key of our meteorology. Both ideas were eagerly followed up—not that there was much to support either, but because they seemed to offer the only possible hope of our being able to foretell the general current of weather change for any long period in advance. So far, however, the first idea may be considered as completely discredited. As to the second, there would appear to be, in the case of certain great tropical and continental countries like India, some slight but by no means conclusive evidence of a connection between the changes in the annual rainfall and the changes in the spotted surface of the sun. Dr. Meldrum, the late veteran Director of the great Meteorological Observatory in Mauritius, has expressed himself as confident that the years of most spots are the years of most violent cyclones in the Indian Ocean. But this is about as far as real progress has been made, and it may be taken as certain that many years more of observation will be required, and the labours of many skilful investigators, before we can hope to carry much farther our knowledge as to any connection between storm and sun.
A further relation of great interest has come to light within the last few years. The year 1868 opened a new epoch in the study of eclipses of the sun. These, perhaps, scarcely lie within the scope[264] of a book on the Royal Observatory, since Greenwich has seen but one in all its history. That fell in the year 1715; for the next it must wait many centuries. Yet, as the late Astronomer Royal conducted three expeditions to see total eclipses, and as the present Astronomer Royal has undertaken a like number, and members of the staff have been sent on other occasions, it may not be deemed quite a digression to refer to one feature which they have brought to light.
When the dark body of the moon has entirely hidden the sun, we have revealed to us, there and then only, that strange and beautiful surrounding of the sun which we call the corona. The earlier observations of the corona seem to reveal it as a body of the most weird and intricate form, a form which seemed to change quite lawlessly from one eclipse to another. But latterly it has been abundantly clear that the forms which it assumes may be grouped under a few well-defined types. In 1878 the corona was of a particularly simple and striking character. Two great wings shot out east and west in the direction of the sun's equator; round either pole was a cluster of beautiful radiating 'plumes.' It was then recollected that the corona of 1867 had been of precisely the same character, both years being years when sun-spots were at their fewest. The coronæ, on the other hand, seen at times when sun-spots are more abundant, were of an altogether different character, the streamers being irregularly distributed all round the sun. Other types also have been recognized, and it is perfectly apparent that the[265] corona changes its shape in close accordance with the eleven-year period. The eclipses of 1889 and 1900, for example, showed coronæ that bore the very closest resemblance to those of 1878 and 1866, the interval of eleven years bringing a return to the same form.
The further problem, therefore, now confronts us: Does the corona produce the sun-spots, or do the sun-spots produce the corona, or are both the result of some mysterious magnetic action of the sun, an action powerful enough on occasion to thrill through and through this world of ours, ninety-three millions of miles away?
CHAPTER XI
THE SPECTROSCOPIC DEPARTMENT
Another department was set on foot by Airy at the same time as the Heliographic Department, and in connection with it; and it is the department which has the greatest of interest for the general public. This deals with astronomical physics, or astrophysics, as it is sometimes more shortly called; the astronomy, that is, which treats of the constitution and condition of the heavenly bodies, not with their movements.
The older astronomy, on the other hand, confined itself to the movements of the heavens so entirely that Bessel, the man whose practical genius revolutionized the science of observation, and whose influence may be traced throughout in Airy's great reconstitution of Greenwich Observatory, denied that anything but the study of the celestial movements had a right to the title of astronomy at all. Hardly more than sixty years ago he wrote:
'What astronomy is expected to accomplish is evidently at all times the same. It may lay down rules by which the movements of the celestial bodies, as they appear to us upon the earth, can be computed. All else which we may learn respecting these bodies, as, for example, their[267] appearance, and the character of their surfaces, is, indeed, not undeserving of attention, but possesses no proper astronomical interest. Whether the mountains of the moon are arranged in this way or in that is no further an object of interest to astronomers than is a knowledge of the mountains of the earth to others. Whether Jupiter appears with dark stripes upon its surface, or is uniformly illuminated, pertains as little to the inquiries of the astronomer; and its four moons are interesting to him only for the motions they have. To learn so perfectly the motions of the celestial bodies that for any specified time an accurate computation of these can be given—that was, and is, the problem which astronomy has to solve.'
There is a curious irony of progress which seems to delight in falsifying the predictions of even master minds as to the limits beyond which it cannot advance. Bessel laid down his dictum as to the true subjects of astronomical inquiry, Comte declared that we could never learn what were the elements of which the stars were composed, at the very time that the first steps were being taken towards the creation of a research which should begin by demonstrating the existence in the heavenly bodies of the elements with which we are familiar on the earth, and should go on to prove itself a true astronomy, even in Bessel's restricted sense, by supplying the means for determining motion in a direction which he would have thought impossible—that is to say, directly to or from us.
The years that followed Kirchhoff's application of the spectroscope to the study of the sun, and his demonstration that sodium and iron existed in the solar atmosphere, were crowded with a succession of brilliant discoveries in the same field. Kirchhoff,[268] Bunsen, Angström, Thal·n, added element after element to the list of those recognized in the sun. Huggins and Miller carried the same research into a far more difficult field, and showed us the same elements in the stars. Rutherfurd and Secchi grouped the stars according to the types of their spectra, and so laid the foundations of what may be termed stellar comparative anatomy. Huggins discovered true gaseous nebulæ, and so revived the nebular theory, which had been supposed crushed when the great telescope of Lord Rosse appeared to have resolved several portions of the Orion nebula into separate stars. The great riddle of 'new stars'—which still remains a riddle—was at least attacked, and glowing hydrogen was seen to be a feature in their constitution. Glowing hydrogen, again, was, in the observation of total eclipses, seen to be a principal constituent of those surroundings of our own sun which we now call prominences and chromosphere. Then the method was discovered of observing the prominences without an eclipse, and they were found to wax and wane in more or less sympathy with the solar spots. Sun-spots, planets, comets, meteors, variable stars, all were studied with the new instrument, and all yielded to it fresh and valuable, and often unexpected, information.
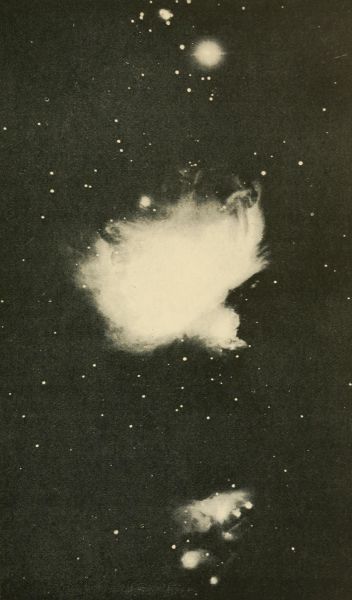
THE GREAT NEBULA IN ORION.
(From a photograph taken at the Royal Observatory Greenwich, December 1, 1899, with an exposure of 21/4 hours.)
In this activity Greenwich Observatory practically took no part. Airy, ever mindful of the original purpose of the Observatory, and deeply imbued with views similar to those which we have quoted from Bessel, considered that the new science lay outside the scope of his duties, until in Mr., now Sir William,
[271] Huggins's skilful hands the spectroscope showed itself not only as a means for determining the condition and constitution of the stars, but also their movements—until, in short, it had shown itself as an astronomical instrument even within Bessel's narrow definition.
The principle of this inquiry is as follows: If a source of light is approaching us very rapidly, then the waves of light coming from it necessarily appear a little shorter than they really are, or, in other words, that light appears to be slightly more blue—the blue waves being shorter than the red—than it really is. A similar thing with regard to the waves of sound is often noticed in connection with a railway train. If an express train, the whistle of which is blowing the whole time, dashes past us at full speed, there is a perceptible drop in the note of the whistle after it has gone by. The sound waves as it was coming were a little shortened, and the whistle therefore appeared to have a sharper note than it had in reality. And in the same way, when it had gone by, the sound waves were a little lengthened, making the note of the whistle appear a very little flatter.
Such a change of colour in a star could never have been detected without the spectroscope; but since when light passes through a prism the shorter waves are refracted more strongly, that is to say, are more turned out of their course than the longer, the spectroscope affords us the means of detecting and measuring this change. Let us suppose that the lines of hydrogen are recognized in a given star. If we compare the spectrum of this star with the spectrum of a tube containing hydrogen and through[272] which the electric spark is passing, we shall be able to see whether any particular hydrogen line occupies the same place as shown by the two spectra. If the line from the star is a little to the red of the line from the tube, the star must be receding from us; if to the blue, approaching us. The amount of displacement may be measured by a delicate micrometer, and the rate of motion concluded from it.
The principle is clear enough. The actual working out of the observation was one of very great difficulty. The movements of the stars towards us, or away from us, are, in general, extremely slow as compared with the speed of light itself; and hence the apparent shift in the position of a line is only perceptible when a very powerful spectroscope is used. This means that the feeble light of a star has to be spread out into a great length of spectrum, and a very powerful telescope is necessary. The work of observing the motions of stars in the line of sight was started at Greenwich in 1875, the 'Great Equatorial' being devoted to it. This telescope, of 123/4 inches aperture, was not powerful enough to do much more than afford a general indication of the direction in which the principal stars were moving, and to confirm in a general way the inference which various astronomers had found, from discussing the proper motions of stars, that the sun and the solar system were moving towards that part of the heavens where the constellations Hercules and Lyra are placed. In 1891, therefore, the work was discontinued, and as already mentioned, the 123/4 telescope by Merz was removed to make room for the present much larger instrument
[275] by Sir Howard Grubb, upon the same mounting. The new telescope being much larger than the one for which mounting and observing room were originally built, it was not possible to put the spectroscope in the usual position, in the same straight line as the great telescope. It was therefore mounted under it, and parallel to it, and the light of the star was brought into it after two reflections. The observer therefore stood with his back to the object and looked down into the spectroscope. It had, however, become apparent by this time that this most delicate field of work was one for which photography possessed several advantages, and as Sir Henry Thompson had made the munificent gift to the Observatory of a great photographic equatorial, it was resolved to devote the 28-inch telescope chiefly to double-star work, and to transfer the spectroscope to the 'New Building.'
The 'New Observatory' in the south ground is crowned indeed with the dome devoted to the great Thompson photographic refractor, but this is not its chief purpose. Its principal floor contains four fine rooms which are used as 'computing rooms'—for the office work, that is to say, of the Observatory. Of these the principal is in the north wing, where the main entrance is placed, and is occupied by the Astronomer Royal and the two chief assistants. The basement contains the libraries and the workshops of the mechanics and carpenters. The upper floor will eventually be used for the storage of photographs and manuscripts, and the terrace roofs of the four wings will be exceedingly convenient for occasional[276] observations, as, for example, of meteor showers. The central dome, which rises high above the level of the terraces, is the only room in the building devoted to telescopic work. As in the New Altazimuth building, a ring of circular lights just below the coping of the wall recalls the portholes of a ship, and again reminds us of the connection of the Observatory with navigation.
Here the spectroscope is now placed, but not, as it happens, on the Thompson refractor. The equatorial mounting in this new dome is a modification of what is usually called the 'German' form of mounting—that is to say, there is but one pier to support the telescope, and the telescope rides on one side of the pier and a counterpoise balances it on the other
[279]The 'Great Equatorial,' on the other hand, is an example of the English mounting, and has two piers, one north and the other south, whilst the telescope swings in a frame between them. In the new dome three telescopes are found rigidly connected with each other on one side of the pier, the telescopes being (1) the great Thompson photographic telescope, double the aperture and double the focal length of the standard astrographic telescope used for the International Photographic Survey; (2) the 123/4 telescope by Merz, that used to be in the great South-East dome, but which is now rigidly connected with the Thompson refractor as a guide telescope; and (3) a photographic telescope of 9 inches aperture, already described as the 'Thompson' photo-heliograph, and used for photographing the sun or in eclipse expeditions. The counterpoise to this collection of instruments is not a mere mass of lead, but a powerful reflector of 30 inches' aperture, and it is to this telescope that the spectroscope is now attached. At the present time, however (August, 1900), regular work has not been commenced with it.
Beside this attempt to determine the motions of the stars as they approach us or retreat from us, on rare occasions the spectroscope has been turned on the planets. As these shine by reflected light, their spectra are normally the same as that of the sun. Mars appeared to the writer, as to Huggins and others, to show some slight indication of the presence of water vapour in its atmosphere. Jupiter and Saturn show that their atmospheres contain some absorbing vapour unknown to ours. And Uranus[280] and Neptune, faint and distant as they are, not only show the same dark band given by the two nearer planets, but several others. More attractive has been the examination of the spectra of the brighter comets that have visited us. The years 1881 and 1882 were especially rich in these. The two principal comets of 1881 were called after their respective discoverers, Tebbutt's and Schaeberle's. They were not bright enough to attract popular attention, though they could be seen with the naked eye, and both gave clear indications of the presence of carbon, their spectra closely resembling that of the blue part of a gas or candle flame. There was nothing particularly novel in these observations, since comets usually show this carbon spectrum, though why they should is still a matter for inquiry; but the two comets of the following year were much more interesting. Both comets came very near indeed to the sun. The earlier one, called from its discoverer Comet Wells, as it drew near to the sun, began to grow more and more yellow, until in the first week of June it looked as full an orange as even the so-called red planet, Mars. The spectroscope showed the reason of this at a glance. The comet had been rich in sodium. So long as it was far from the sun the sodium made no sign, but as it came close to it the sodium was turned into glowing vapour under the fierce solar heat. And as the writer saw it in the early dawn of June 7, the comet itself was a disc of much the same colour as Mars, whilst its spectrum resembled that of a spirit lamp that has been plentifully fed with carbonate of soda or common salt. The 'Great Comet' of the autumn of the same[281] year, and which was so brilliant an object in the early morning, came yet nearer to the sun, and the heating process went on further. The sodium lines blazed up as they had done with Comet Wells, but under the fiercer stress of heat to which the Great Comet was subjected, the lines of iron also flashed out, a significant indication of the tremendous temperature to which it was exposed.
There are two other departments of spectroscopic work which it was attempted for a time to carry on as part of the Greenwich routine. These were the daily mapping of the prominences round the sun, and the detailed examination of the spectra of sun-spots. Both are almost necessary complements of the work done in the heliographic department—that is to say, the work of photographing the appearance of the sun day by day, and of measuring the positions and areas of the spots. For the spots afford but one index out of several, of the changes in the sun's activity. The prominences afford another, nor can we at the present moment say authoritatively which is the more significant. Then again, with regard to the spots themselves, it is not certain that either their extent or their changes of appearance are the features which it is most important for us to study. We want, if possible, to get down to the soul of the spot, to find out what makes one spot differ from another; and here the spectroscope can help us. Great sun-spots are often connected with violent agitation of the magnetic needles, and with displays of auroræ. But they are not always so, and the inquiry, 'What makes them to differ?' has been made again and again,[282] without as yet receiving any unmistakable answer. The great spot of November, 1882, which was connected with so remarkable an aurora and so violent a magnetic storm, was as singular in its spectrum as in its earthly effects. The sun was only seen through much fog, and the spectrum was therefore very faint, but shooting up from almost every part of its area, except the very darkest, were great masses of intensely brilliant hydrogen, evidently under great pressure. The sodium lines were extremely broadened, and on November 20 a broad bright flame of hydrogen was seen shooting up at an immense speed from one edge of the nucleus. A similar effect—an outburst of intensely luminous hydrogen—has often been observed in spots which have been accompanied by great magnetic storms; and it may even be that it is this violent eruption of intensely heated gas which has the directest connection with the magnetic and auroral disturbances here upon earth.
This sun-spot work was not carried on for very long, as only one assistant could be spared for the entire solar work of whatever character. Yet in that time an interesting discovery was made by the writer—namely, that in the green part of the spectrum of certain spots a number of broad diffused lines or narrow bands made their appearance from time to time, and especially when sun-spots were increasing in number, or were at their greatest development.
The prominence work had also to be dropped, partly for the same reason, but chiefly because the atmospheric conditions at Greenwich are not suitable for these delicate astrophysical researches. When[283] the Observatory was founded 'in the golden days' of Charles II., Greenwich was a little country town far enough removed from the great capital, and no interference from its smoke and dust had to be feared or was dreamt of. Now the 'great wen,' as Cobbett called it, has spread far around and beyond it, and the days when the sky is sufficiently pure round the sun for successful spectrum work on the spots or prominences are few indeed.
Whether in the future it will be thought advisable for the Royal Observatory to enter into serious competition in inquiries of this description with the great 'astrophysical' observatories of the Continent and of America—Potsdam, Meudon, the Lick, and the Yerkes—we cannot say. That would involve a very considerable departure from its original programme, and probably also a departure from its original site. For the conditions at Greenwich tend to become steadily less favourable for such work, and it would most probably be found that full efficiency could only be secured by setting up a branch or branches far from the monster town.
With the older work it is otherwise. So long as Greenwich Park and Blackheath are kept—as it is to be hoped they always will be—sacred from the invasion of the builder; so long as no new railways burrow their tunnels in the neighbourhood of the Observatory, so long the fundamental duties laid upon Flamsteed, 'of Rectifying the Tables of the Motions of the Heavens and the Places of the Fixed Stars,' will be carried out by his successors on Flamsteed Hill.
CHAPTER XII
THE ASTROGRAPHIC DEPARTMENT
The two last departments mentioned, the heliographic and spectroscopic, lie clearly and unmistakably outside the terms of the original warrant of the Observatory, though the progress of science has led naturally and inevitably to their being included in the Greenwich programme. But the Astrographic Department, though it could no more have been conceived in the days of Charles II. than the spectroscopic, does come within the terms of the warrant, and is but an expansion of that work of 'Rectifying the Places of the Fixed Stars,' which formed part of the programme enjoined upon Flamsteed, the first Astronomer Royal, at the first foundation of the Observatory, and which was so diligently carried out by him, the first Greenwich catalogue, containing about 3000 stars, being due to his labours.
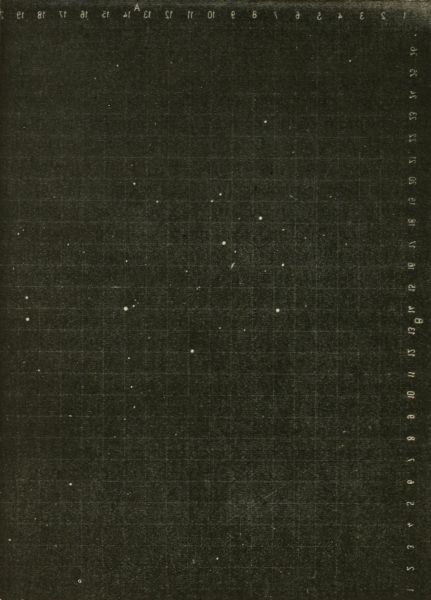
'CHART PLATE' OF THE PLEIADES.
(From a photograph taken at the Royal Observatory, Greenwich, with an exposure of forty minutes.)
His immediate successors did much less in this field, though Bradley's observations were published, long after his death, as a catalogue of 3222 stars, in some aspects the most important ever issued. Pond, the sixth Astronomer Royal, restored catalogue-making to a prominent place in the Greenwich routine,
[287] and his precedent is sedulously followed to-day. But each of these was confined to about 3000 stars. The necessity has long been felt for a much ampler census, and Argelander, at the Bonn Observatory, brought out a catalogue of 324,000 stars north of South declination 2°, a work which has been completed by Schönfeld, who carried the census down to South declination 23°, and by the two great astronomers of Cordoba, South America, Dr. Gould and Dr. Thome, by whom it was extended to the South Pole.
These last three catalogues embrace stars of all magnitudes down to the 9th or 10th; but certain astronomers had endeavoured to go much lower, and to make charts of limited portions of the sky down to even the 14th magnitude.
From the very earliest days that men observed the stars, they could not help noticing that 'one star differeth from another star in glory,' and consequently they divided them into six classes, according to their brightness—classes which are commonly spoken of now as magnitudes. The ordinary 6th magnitude star is one which can be clearly seen by average sight on a good night, and it gives us about one-hundredth the light of an average 1st magnitude star. Sirius, the brightest of all the fixed stars, is called a 1st magnitude star, but is really some six or seven times as bright as the average. It would take, therefore, more than two and a half million stars of the 14th magnitude to give as much light as Sirius.
It is evident that so searching a census as to embrace stars of the 14th magnitude would involve a most gigantic chart. But the work went on in[288] more than one Observatory for a considerable time, until at last the observers entered on to the region of the Milky Way. Here the numbers of the stars presented to them were so great as to baffle all ordinary means of observation. What could be done?
Just at this time immense interest was caused in the astronomical world by the appearance of the great comet of 1882. It was watched and observed and sketched by countless admirers, but more important still, it was photographed, and some of its photographs, taken at the Royal Observatory, Cape of Good Hope, showed not only the comet with marvellous beauty of detail, but also thousands of stars, and the success of these photographs suggested to her Majesty's Astronomer at the Cape, Dr. Gill, that in photography we possessed the means for making a complete sky census even to the 14th magnitude.
The project was thought over in all its bearings, and in 1887 a great conference of astronomers at Paris resolved upon an international scheme for photographing the entire heavens. The work was to be divided between eighteen Observatories of different nationalities. It was to result in a photographic chart extending to the 14th magnitude, and probably embracing some forty million stars, and a catalogue made from measures of the photographs down to the 11th magnitude, which would probably include between two and three million stars.
The eighteen Observatories all undertook to use instruments of the same capacity. This was to be a photographic refractor, with an object-glass of 13[289]
[290] inches aperture and 11 feet focus. At Greenwich this telescope is mounted equatorially—that is, so as to follow the stars in their courses—and is mounted on the top of the pier that once supported Halley's quadrant. The telescope is driven by a most efficient clock, whose motive power is a heavy weight. The rate of the weight in falling is regulated by an ingenious governor, which brings its speed very nearly indeed to that of the star, and any little irregularities in its motion are corrected by the following device. A seconds pendulum is mounted in a glass case on the wall of the Observatory, and a needle at the lower end of the pendulum passes at each swing through a globule of mercury. On one of the wheels of the clock are arranged a number of little brass points, at such intervals apart that the wheel, when going at the proper rate, takes exactly one second to move through the distance between any pair. A little spring is arranged above the wheel, so that these points touch it as they pass. If this occurs exactly as the pendulum point passes through the mercury nothing happens, but if the clock is ever so little late or early, the electric current from the pendulum brings into action a second wheel, which accelerates or retards the driving of the clock, as the case may be. The total motion, therefore, is most beautifully even.
But even this is not quite sufficient, especially as the plates for the great chart have to be exposed for at least forty minutes. Rigidly united with the 13-inch refractor, so that the two look like the two barrels of a huge double-barrelled gun, is a second[291] telescope for the use of the observer. In its eyepiece are fixed two pairs of cross spider lines, commonly called wires, and a bright star, as near as[292] possible to the centre of the field to be photographed, is brought to the junction of two wires. Should the star appear to move away from the wire, the observer has but to press one of two buttons on a little plate which he carries in his hand, and which is connected by an electric wire with the driving clock, to bring it back to its position.
The photographs taken with this instrument are of two kinds. Those for the great chart have but a single exposure, but this lasts for forty minutes. Those for the great catalogue have three exposures on them, the three images of a star being some 20 seconds of arc apart. These exposures are of six minutes', three minutes', and twenty seconds' duration, and the last exposure is given as a test, since, if stars of the 9th magnitude are visible with an exposure of twenty seconds, stars of the 11th magnitude should be visible with three minutes' exposure.
Thus it will be seen that in three minutes an impression is got of many scores of stars, whose places it would require many hours to determine at the transit instrument. But the positions of these stars on the plate still remain to be measured. For this purpose a net-work of lines, at right angles to each other, is printed on the photograph before its development, and, after it has been developed, washed and dried, the distances of the stars from their nearest cross-lines are measured in the measuring machine.
The measuring machine is constructed to hold two plates, one half its breadth higher than the other.
[294]
[295] In fact, in each of the two series of photographs the whole sky is taken twice, but the two photographs of any region are not simply duplicates of each other. The centre of each plate is at a corner of four other plates, and in the micrometer the stars on the quarter common to two plates are measured simultaneously.
In this way will be carried out a great census of the sky that will exceed Flamsteed's ten thousand fold. And just as Flamsteed's was but the first of many similar catalogues, so, no doubt, will this be followed by others—not superseded, for its value will increase with its age and the number of those that follow it, by comparison with which it will prove an inexhaustible mine of information concerning the motions of the stars and the structure of the universe.
There is a great difference between the work of the observer with the 'Astrographic Telescope,' as this great twin photographic instrument is called, and the work of the transit observer. The latter sees the star gliding past him, and telegraphs the instant that the star threads itself on each of the ten vertical wires in succession. The astrographic observer, on the other hand, sees his star shining almost immovably in the centre of his field, threaded on the two cross wires placed there, for the driving-clock moves the telescope so as to almost exactly compensate for the rotation movement of the earth. The observer's duty in this case is to telegraph to his driving-clock, when it has in the least come short of or exceeded its duty, and so to bring back the[296] 'guiding star' to its exact proper place on the cross wires.
So far, the work of the Astrographic Department has been, as mentioned above, a development on an extraordinary scale, but a development still, of the original programme of the Observatory. But the munificent gift of Sir Henry Thompson has put it within the power of the Astronomer Royal to push this work of sidereal photography a stage further. Sir Henry Thompson gave to the Observatory, not merely the photographic refractor of 9 inches' aperture, now used for solar photography, and known as the 'Thompson photo-heliograph,' but also one of 26 inches' aperture and 221/2 feet focal length. This instrument was specially designed of exactly double the dimensions of the standard astrographic telescope used for the International Photographic Survey, the idea being that, in the case of a field of special interest and importance, a photograph could be obtained with the larger instrument on exactly double the scale given by the smaller. It has rather, however, found its usefulness in a slightly different field. The observation of the satellites of Jupiter was suggested by Galileo as a means of determining the longitude at sea. As already pointed out, the suggestion did not prove to be a practical one for that purpose, but observations of the satellites have been made none the less with a view simply to improving our knowledge of their movements, and of the mass of Jupiter. The utilitarian motive for the work having fallen through, it has been carried on as a matter of pure science.
And the work has not stopped with the satellites of Jupiter; eight satellites were in due time discovered to Saturn, four to Uranus, and two to Mars; and though these could give not the remotest assistance to navigation, they too have been made the subjects of observation for precisely the same reason as those of Jupiter have been.
In just the same way, when the discovery of Neptune was followed by that of a solitary companion to it, this also had to be followed. The difficulties in the way of observing the fainter of all these satellites were considerable, and the work has[298] been mostly confined to two or three observatories possessing very large telescopes. As the largest telescope at Greenwich was only 7 inches in aperture up to 1859, and only 123/4 inches up to 1893, it is only very recently that it has been able to take any very substantial part in satellite measures. But since the Thompson photographic telescope was set up, it has been found that a photograph of Neptune and its satellite can be taken in considerably less time than a complete set of direct measures can be made, whilst the photograph, which can be measured at leisure during the day, gives distinctly the more accurate results.
So, too, the places of the minor planets can be got more accurately and quickly by means of photographs with this great telescope than by direct observation, and photographs of the most interesting of them all, the little planet Eros, have been very successfully obtained. So that, though doing nothing directly to improve the art of navigation, or to find the longitude at sea, the great photographic refractor takes its share in the work of 'Rectifying the Tables of the Planets.'
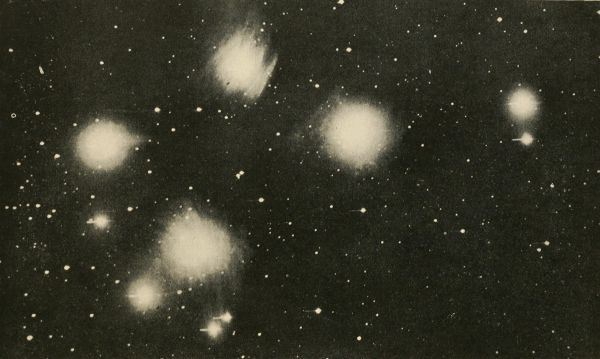
THE NEBULÆ OF THE PLEIADES.
(From a photograph taken at the Royal Observatory, Greenwich, December 3, 1899, with an exposure of three hours.)
The reflector of 30 inches' aperture, which acts as a counterpoise to the sheaf of telescopes of the Thompson, is intended for use with the spectroscope, the quality which mirrors possess of bringing all rays, whatever their colour, to the same focus being of great importance for spectroscopic work. But the experiments which have been made with it in celestial photography have proved so extremely successful as to cause the postponement of the recommencement
[301] of the spectroscopic researches. Chief amongst these photographs are some good ones of the moon, and more recently some exceedingly fine photographs of the principal nebulæ.
In no department of astronomy has photography brought us such striking results as in regard to the nebulæ. Dr. Roberts' photograph of the great nebula in Andromeda converted the two or three meaningless rifts—which some of the best drawings had shown—into the divisions between concentric rings; and what had appeared a mere shapeless cloud was seen to be a vast symmetrical structure, a great sidereal system in the making. The great nebula in Orion has grown in successive photographs in detail and extent, until we have a large part of the constellation bound together in the convolutions of a single nebula of the most exquisite detail and most amazing complexity. The group of the Pleiades has had a more wonderful record still. Manifestly a single system even to the naked eye, and showing some faint indications of nebulosity in the telescope, the photographs have revealed its principal stars shining out from nebulous masses, in appearance like carded wool, and have shown smaller stars threaded on nebulous lines like pearls upon a string.
Such photographs are, of course, of no utilitarian value, and at present they lead us to no definite scientific conclusions. They lie, therefore, doubly outside the limits of the purely practical, but they attract us by their extreme beauty, and by the amazing difficulty of the problems they suggest. How are these weird masses of gas retained in such[302] complex form over distances which must be reckoned by millions of millions of miles? By what agency are they made to glow so as to be visible to us here? What conceivable condition threads together suns on a line of nebula? What universes are here in the making, or perhaps it may be falling into ruin and decay?
CHAPTER XIII
THE DOUBLE-STAR DEPARTMENT
The foregoing chapters will have shown that though the original purpose of the Observatory has always been kept in view, yet the progress of science has caused many researches to be undertaken which overstep its boundaries. Thus in the present transit room, beside the successive transit instruments we find upon the wall two long thin tubes, labelled respectively Alpha Aquilæ and Alpha Cygni. These were two telescopes set up by Pond for a special purpose. Dr. Brinkley, Royal Astronomer for Ireland, had announced that he had found that several stars shifted their apparent place in the sky in the course of a year, due to the change in the position of the earth from which we view them, by an amount which would show that they were only about six to nine billions of miles distant from us; or, in other words, they showed a parallax of from two to three seconds of arc. Pond was not able to confirm these parallaxes from his observations, and to decide the point he set up these two telescopes, the Alpha Aquilæ telescope being rigidly fixed on the west side of the pier of Troughton's mural circles; the Alpha[304] Cygni telescope on another pier, the one which now forms the base of the pier of the astrographic telescope. Pond's method was to compare the position of these two stars with that of a star almost exactly the same distance from the pole, but at a great distance from it in time of crossing the meridian; in other words, of almost the same declination, but widely different right ascension. The result proved that Brinkley was wrong, and vindicated the delicacy and accuracy of Pond's observations.
These two telescopes, therefore, had their day and ceased to be. Others have followed them. An ingenious telescope was set up by Sir George Airy in order to ascertain if the speed of light were different when passing through water than when passing through air. Or, in other words, if the aberration of light would give the same value as at present if we observed through water. The water telescope, as it was called, is kept on the ground floor of the central octagon of the new observatory. The observations obtained with it were hardly quite satisfactory, but gave on the whole a negative result.
Turning back to the transit room, and leaving it by the south-west door, we come into the little passage which leads at the back of Bradley's transit room into the lower computing room. Just inside this passage, on the left-hand side, there is a little room of a most curious shape, the 'reflex zenith room.' Here is fixed a telescope pointing straight upwards, the eye-piece being fixed by the side of the object-glass. The light from a star—the star Gamma Draconis—which passes exactly over the zenith of[305] Greenwich, enters the object-glass, passes downwards to a basin of mercury, and is reflected upwards from the surface of the mercury to a little prism placed over the centre of the object-glass, from which it is reflected again into the eye-piece. By means of this telescope the distance of the star Gamma Draconis from the zenith could be measured very exactly, and, consequently, the changes in the apparent position of the star due to aberration, parallax, and other causes could be very exactly followed, and the corrections to be applied on account of these causes precisely determined.
This particular telescope was devised by Airy, and the observations with it were continued to the end of his reign. The germ of the idea may be traced back, however, to the time of Flamsteed, who would seem to have occasionally observed Gamma Draconis from the bottom of a deep well; the precise position of the well is not, however, now known. Later, Bradley set up his celebrated 121/2-foot zenith sector, still preserved in the transit room, first at Wanstead and then at Greenwich, for the determination of the amount of aberration. Later, a zenith tube by Troughton, of 25 feet focus, was used by Pond in conjunction with the mural circle for observations of Gamma Draconis in order to determine the zenith point of the latter instrument.
These telescopes for special purposes have passed out of use. Observations with the spectroscope have been suspended for some years. The work of the Astrographic Department will come to an end, in the ordinary course of events, when the programme[306] assigned to Greenwich in the International Scheme is completed.
Within the last few years a new department has come into being at Greenwich—a department which has been steadily worked at many foreign public observatories, but only recently here.
This is the Department of Double-Star Observation. The first double star, Zeta Ursæ Majoris, was discovered 250 years ago. Bradley discovered two exceedingly famous double stars whilst still a young man observing with his uncle at Wanstead—Gamma Virginis and Castor. Bradley made also other discoveries of double stars after his appointment to Greenwich, and Maskelyne succeeded him in the same line, but the great foundation of double-star astronomy was laid by Sir William Herschel.
At first it was supposed that double stars were double only in appearance; one star comparatively near us 'happened' to lie in almost exactly the same direction as another star much further off. It was, indeed, in the very expectation that this would prove to be the case, that the elder Herschel first took up their study. But he was soon convinced that many of the objects were true double stars—members of the same system of which the smaller revolved round the larger—not merely apparently double, one star appearing by chance to be close to another with which it had no connection—but real double stars. The discovery of these has led to the establishment of a new department of astronomy, again scientific rather than utilitarian.
As mentioned above, it is only recently that
[309]Greenwich has taken any appreciable part in this work. Under Airy, the largest equatorial of the time had been furnished with a good micrometer, and observations of one or two double stars been made now and again; but Airy's programme of work was far too rigid, and kept the staff too closely engaged for such observations to be anything but extremely rare. And, indeed, when the micrometers of the equatorials were brought into use, they were far more generally devoted to the satellites of Saturn than to the companions of stars. In the main, double-star astronomy has been in the hands of amateurs, at least in England. But the discovery in recent years of many pairs so close that a telescope of the largest size is required for their successful observation, has put an important section of double stars beyond the reach of most private observers, and therefore the great telescope at Greenwich is now mainly devoted to their study. The Astronomer Royal, therefore, soon after the completion of the great equatorial of 28-inches aperture placed in the south-east dome, added this work to the Observatory programme.
The 28-inch equatorial is a remarkable-looking instrument, its mounting being of an entirely different kind to that of the other equatorials in the Observatory, with the solitary exception of the Shuckburgh, which is set up in a little dome over the chronograph room. The Shuckburgh was presented to the Observatory in the year 1811, by Sir G. Shuckburgh. It was first intended to be mounted as an altazimuth, but proved to be unsteady in that position, and was then converted into an equatorial without clockwork,[310] and mounted in its present position. The position is about as hopelessly bad a one as a telescope could well have, completely overshadowed as it is by the trees and buildings close at hand. The dome is a small one, and the arrangements for the shutters and for turning the dome are as bad as they could possibly be. It has practically been useless for the last forty years.
Its only interest is that the method of mounting employed is a small scale model of that of the great telescope in the S.-E. dome. In the German or Fraunhofer form of mounting for an equatorial there is but a single pillar, which carries a comparatively short polar axis. At the upper end of the polar axis we find the declination axis, and at one end of the declination axis is the telescope, whilst at the other end is a heavy weight to counterpoise it. The German mounting has the advantage that the telescope can easily point to the pole of the heavens; its drawbacks are that, except in certain special forms, the telescope cannot travel very far when it is on the same side of the meridian as the star to which it is pointed, the end of the telescope coming into contact under such circumstances with the central pier, whilst the introduction of mere deadweight as the necessary counterpoise, is not economical. It has been already pointed out that the present Astronomer Royal has not only considerably modified the German mounting in the great collection of telescopes in the Thompson dome, but has used a powerful reflector as a counterpoise to the sheaf of refractors at the other end of the declination axis.
The English equatorial requires two piers.[311] Between these two piers is a long polar axis. Both in the little Shuckburgh and in the great 28-inch equatorial the frame of the polar axis consists of six parallel rods disposed in two equilateral triangles, with their bases parallel to each other, the telescope swinging in the space between the two bases. The construction of this form of equatorial, therefore, is expensive, as it requires two piers. It takes much more room than the German form, and the telescope cannot be directed precisely to the pole. But the instrument is symmetrical, there is no deadweight, and the telescope can follow a star from rising to setting without having to be reversed on crossing the meridian.
The great stability of the English form of mounting, therefore, commended it very highly to Airy, and he designed the great Northumberland equatorial of the Cambridge Observatory on that plan, as well as one for the Liverpool Observatory at Bidston, and in 1858 the S.-E. equatorial at Greenwich.
The telescope at first mounted upon it had an object-glass of 123/4 inches' aperture, and 18 feet focal length. That was dismounted in 1891, and is now used as the guiding telescope of the Thompson 26-inch photographic refractor. Its place was taken by an immensely heavier instrument, the present refractor of 28 inches' aperture, and 28 feet focal length; and that this change was effected safely was an eloquent testimony to the solidity of the original mounting.
The clock that drives this great instrument, so that it can follow a star or other celestial object in its apparent daily motion across the sky, is in the basement of the S.-E. tower. It is a very simple[312] looking instrument, a conical pendulum in a glass case. The pendulum makes a complete revolution once in two seconds. Below it in a closed case is a water turbine. A cistern on the roof of the staircase supplies this turbine with water, having a fall of about thirty feet. The water rushing out of the arms of the turbine forces it backward, and the turbine spins rapidly round, driving a spindle which runs up into the dome, and gears through one or two intermediate wheels with the great circle of the telescope; the extremely rapid rotation of the spindle, four times in a second, being converted by these intermediate wheels into the exceedingly slow one of once in twenty-four hours. Just above the centre of motion of the turbine is a set of three small wheels, all of exactly the same size, and of the same number of teeth. Of these the bottom wheel is horizontal, and is turned by the turbine. The top wheel is also horizontal, and is turned by the pendulum. The third wheel gears into both these, and is vertical. If the top and bottom wheels are moving exactly at the same rate, the intermediate wheel simply turns on its axis, but does not travel; but if the turbine and pendulum are moving at different rates, then the vertical wheel is forced to run in one direction or the other, and, doing so, it opens or closes a throttle valve, which controls the supply of water to the turbine, and so speedily brings the turbine into accord with the pendulum. The control of the motion of the great telescope is therefore almost as perfect as that of the astrographic and Thompson equatorials, though the principle employed is very[313] different. And the control needs to be perfect, for, as said above, the great telescope is mostly devoted to the observation of double stars, and there can be no greater hindrance to this work than a telescope which does not move accurately with the star.
There is a striking contrast between the great telescope and all the massive machinery for its direction and movement, and the objects on which it is directed—two little points of light separated by a delicate hair of darkness.
The observation is very unlike those of which we have hitherto spoken. The object is not to ascertain the actual position in the sky of the two stars, but their relative position to each other. A spider's thread of the finest strands is moved from one star to the other by turning an exquisitely fine screw; this enables us to measure their distance apart. Another spider thread at right angles to the first is laid through the centres of both stars, and a divided circle enables us to read the angle which this line makes to the true east and west direction. Such observations repeated year after year on many stars have enabled the orbits of not a few to be laid down with remarkable precision; and we find that their movements are completely consistent with the law of gravitation. Further, just as Neptune was pre-recognized and discovered from noting the irregularities in the motion of Uranus, so the discordances in the place of Sirius led to the belief that it was attracted by a then unseen companion, whose position with respect to the brighter star was predicted and afterwards seen.
Gravitation thus appears, indeed, to be the Bond[314] of the Universe, yet it leaves us with several weighty problems. The observation of the positions of stars shows that though we call them fixed they really have motions of their own. Of these motions, a great part consists of a drift away from one portion of the[315] heavens towards a point diametrically opposite to it, a drift such as must be due, not to a true motion of the individual stars, but to a motion through space of our sun and its attendant system. The elder Herschel was the first to discover this mysterious solar motion. Sir George Airy and Mr. Edwin Dunkin, for forty-six years a member of the Greenwich staff, and from 1881-1884 the Chief Assistant, contributed important determinations of its direction.
What is the cause of this motion, what is the law of this motion, is at present beyond our power to find out. Many years ago a German astronomer made the random suggestion that possibly we were revolving in an orbit round the Pleiades as a centre. The suggestion was entirely baseless, but unfortunately has found its way into many popular works, and still sometimes is brought forward as if it were one of the established truths of astronomy. We can at present only say that this solar motion is a mystery.
There is a greater mystery still. The stars have their own individual motions, and in the case of a few these are of the most amazing swiftness. The earth in its motion round the sun travels nearly nineteen miles in a second, say one thousand times faster than the quickest rush of an express train. The sun's rate of motion is probably not quite so swift, but Arcturus, a sun far larger than our own, has a pace some twenty times as swift as the orbital motion of the earth. This is not a motion that we can conceive of as being brought about by gravitation, for if there were some unseen body so vast as to draw Arcturus with this swiftness, other stars too[316] would be hurtling across the sky as quickly. Such 'runaway stars' afford a problem to which we have as yet no key, and, like Job of old, we are speechless when the question comes to us from heaven, 'Canst thou guide Arcturus and his sons?'
It will be seen then that, fundamentally, Greenwich Observatory was founded and has been maintained for distinctly practical purposes, chiefly for the improvement of the eminently practical science of navigation. Other inquiries relating to navigation, as, for instance, terrestrial magnetism and meteorology, have been added since. The pursuit of these objects has of necessity meant that the Observatory was equipped with powerful and accurate instruments, and the possession of these again has led to their use in fields which lay outside the domain of the purely utilitarian, fields from which the only harvest that could be reaped was that of the increase of our knowledge. So we have been led step by step from the mere desire to help the mariner to find his way across the trackless ocean, to the establishment of the secret law which rules the movements of every body of the universe, till at length we stand face to face with the mysteries of vast systems in the making, with the intimate structure of the stellar universe, with the apparently aimless, causeless wanderings of vast suns in lightning flight; with problems that we cannot solve, nor hope to solve, yet cannot cease from attempting, problems to which the only answer we can give is the confession of the magicians of Egypt—'This is the finger of God.'
INDEX
Adams, John C., his discovery of Neptune, 217
Adhara, 183
Airy, George Biddell, seventh Astronomer Royal, his early life, 102;
his work at Cambridge, 105;
comes to Greenwich, 105;
his relations with the Visitors, 106;
his autobiography, 108;
his character, 111;
his labours, 113;
attacks on, 114;
his distinctions, 118;
his resignation, 119;
his death, 120;
anecdote of, 142;
his conduct re Adams, 217;
his water telescope, 304
Alderamin, 183
Almagest, 185
Almanac making, 29
Alpha Aquilæ, telescope for, 303
—— Cygni, telescope for, 303
Altazimuth the, 114;
description and work of, 207, et seq.
Altazimuth Department, 205, et seq.
American time, 153
Andromeda nebula, 301
Anemometer, use of, 238;
trace of, 242
Angström, 268
Anson, Commodore, 17
Apparent time, 152
Arcturus, motion of, 315
Argelander, star catalogue of, 287
Art of Dialling, the, 28
Assistants, position of the, 98, 100, 117, 137
Astrographic chart, 128
—— Department, 284, et seq.
—— dome, 128
—— telescope, 289, et seq.
Astronomers Royal, the, 25
Astrophysical researches, 282
Auroræ, 281
Automatic register, 241
Axis of the earth, precession of, 184
Ball, Time, 162
Barometer, use of the, 192, 233
Battery basement, 161
Beaufort, Captain, 107
Bessel quoted, 266
Betelgeuse, 184
Birkenhead, wreck of the, 180
Bliss, Nathaniel, fourth Astronomer Royal, history of, 82
Bradley, James, third Astronomer Royal, his life, 73;
his ordination, 74;
Vicar of Bridstow, 74;
Savilian Professor of Astronomy, 75;
discovers Aberration of Light, 75, et seq.;
becomes Astronomer Royal, 79;
labours of, 80;
character of, 81
Bradley's transit room, 128
Brinkley, Dr., 303
British Mariner's Guide, the, 90
Bunsen, 268
Buys Ballot's law, 237
Canadian time, 153
Castor, 74, 306
Catalogues, star, 182, 185, et seq., 198, 284
Cepheus, 183
Charles II., warrants of, 39, 40
Christie, W. H. M., eighth Astronomer Royal, work of, 120
Chromosphere of the sun, 268
Chronograph, the, 157
—— room, 126
Chronometer business, 101, 107
Chronometers, Harrison's improvements in, 165, et seq.;
tests of, 169;
'runs' of, 173;
romance of, 178
Circle Department, 181, et seq.
Clock, Astrographic driving, 290;
driving 28-inch telescope, 312
Clocks, standard, 160
Columbus, aim of voyage of, 18
Comet, appearance of a, 28
—— Wells, 280
Comets, observation of, 224;
spectra of, 280
Commutator, the, 162
Comte, assertion of, 267
Constant of Aberration, 79
Cook, Captain, work of, 170
Copper, use of in Observatory, 245
Corona of the sun, 264
Crabtree, James, 31
[318]Crosthwait, Joseph, 57
Dallmeyer telescope, 252
Declination, 186, et seq.
Denebola, 184
Distances of planets, 223;
of sun, 224
Double-Star Department, 303, et seq.
Double Stars, 306
Dublin time, 155
Dunkin, Edwin, 315
Earth, the, movements of, 201
Eclipses of the moon, 216;
of the sun, July 25, 1748...85;
other eclipses of the sun, 263, et seq.
Electric Railway, influence of, 249
Equation of Time, the, 29, 151
Equatorial, Shuckburgh's, 101
——, the great 28-inch, 221
——, the Merz, 123/4-inch, 114
——, 28-inch, driving clock of, 309;
use of, 313
——, clock-driven, 74
Eros, discovery of, 223;
photographs of, 298
Errors in observations, noting of, 199, et seq.
Evaporation, 241
Faculæ of the sun, 257
Flamsteed, John, his report on Saint-Pierre's proposal, 23, 32;
appointed first Astronomer Royal, 23, 34;
his autobiography, 26;
his studies, 29;
his almanac, 29;
sent to London, 30;
enters Jesus College, Cambridge, 31;
completes his observatory, 31;
acquaintance with Newton, 31;
takes his degree, 32;
his work, 34;
warrant for his salary, 39;
position of, 42;
his ordination, 45;
his pupils, 45;
his trouble with Newton, 46, et seq.;
his catalogue, 53;
his letter to Sharp, 54;
his death, 56;
his labours, 57
Flamsteed House, 126
Fraunhofer mounting, 310
French time, 155
Galileo, his discovery of Jupiter's satellites, 19
Gamma Draconis, 75, 304
—— Virginis, 306
Gascoigne, William, 31
Gemma Frisius, plan of, 22
George of Denmark, Prince, 50
German mounting, 276, 310
Gould, Dr., 287
Graham, 166
Gravitation, the bond of the universe, 313
Great comet of 1882, the, 280, 288
Greatrackes, Valentine, 29
Green, Charles, 91
Greenwich time, 153;
distribution of, 163
Halley, Edmund, his life, 60;
his early work, 60;
his catalogue of stars, 63;
elected F.R.S., 63;
his work on Kepler's laws, 64;
becomes captain, 65;
Savilian Professor of Geometry, 66;
Astronomer Royal, 66;
observations on saros of the moon, 67;
pressed by Newton, 68;
his death, 68;
his services to science, 68;
his pay, 70;
nominates his successor, 73;
his transit instrument, 73
Halley's comet, 225
Harrison, James, timekeepers of, 86, 91, 93, 165
Heineken, Rev. N. S., 59
Heineken quadrant, 59
Heliographic Department, 251, et seq.
Herschel, Caroline, 57
Hipparchus, catalogue of, 185
Hodgson, Mr., 50
Hooke, Robert, 75, 206
Horrox, Jeremiah, 31
Huggins, Sir W., his use of spectroscope, 268
Inscription, an, 126
International Photographic Survey, 296
Ireis, 224
Iron quadrant, 73
Isobars, 237
Jupiter, satellites of, 19, 296;
atmosphere of, 279
Keill, John, 74
Kendall, Larcum, 166
Kepler, laws of, 64
Kew, photo-heliograph, the, 252
Kinnebrook, David, 176
Kirchhoff's use of spectroscope, 267
Latitude, finding the, 18
Ledgers, chronometer, romance of, 176
Leverrier, his discovery of Neptune, 217
Libraries, 132
Linacre, G., 28
Lindsay, Thomas, quoted, 204
Litchford, W., 28
Local apparent time, 22
Longitude, finding the, 18;
at sea, problem of, 86;
determination of, 173
Longitude nought, 148
Lower computing room, 128
Lunars, method of, 86
Magnetic Department, work of, 133;
description of, 228, et seq.
Magnetic inclination and declination, 246
—— needles, movements of, 247, 262
—— observatory, 132
—— pavilion, 245
—— storms, 248, 262
Mars, distance of, 223;
atmosphere of, 279;
[319]satellites of, 296
Maskelyne, Nevil, fifth Astronomer Royal, 85;
practical work of, 86;
Astronomer Royal, 91;
his work, 92;
his publications, 92;
his observations and work, 92, et seq.;
his death, 94;
his character, 97;
recommends his successor, 97;
his mural circle, 101
Mean solar clock, 160
Mean time, 152
Meldrum, Dr., on sun spots, 263
Meridian, the, 149
Merz telescope, 279
Meteorological Department, work of, 133;
description of, 228, et seq.
Micrometers, use of, 309
Microscopes, use of, 188
Milky Way, 288
Miller, Professor, 268
Milne, Professor, on earth movements, 201
Minor planets, 222
Molyneux, Samuel, 75
Moon, observation of the, 212, et seq.;
eclipses of, 266
Moore, Sir Jonas, 30;
death of, 42
Morin, 33
Mounting telescopes, modes of, 310
Mudge, Thomas, 94
Mural arc, 7-feet, 46
Mural circles, 101, 196
Names of stars, origin of, 183
Nares, Sir George, 170
Nautical Almanac, the, 22, 23, 92
Navigation, state of primitive, 17
Neptune, discovery of, 217;
atmosphere of, 280;
satellite of, 298
New altazimuth, the, 132, 210
New Observatory, the, 136, 275
New stars, 268
Newcomb, Professor, on growth of Observatory, 124;
on Greenwich observations, 207
Newton, Sir I., his absent-mindedness, 31;
his trouble with Flamsteed, 46, et seq.;
on Kepler's laws, 65;
his Principia, 65;
his pressure on Halley, 68;
his discovery of gravitation, 206
North terrace, the, 126
Northumberland equatorial, 218
Nutation of the earth, 80
Observation, modes of, 156, 176, 188;
by reflection, 196;
of comets, 224
Observatory, Greenwich, work of, 13;
foundation of, 23;
warrant for building, 40;
position of, 41;
foundation stone laid, 42;
condition of, 79;
enlargement of, 112;
recent extensions of, 120;
description of, 124, et seq.;
staff of, 137;
work of, 139, et seq.;
visitors to, 175;
new altazimuth building, 211;
magnet house, 228;
magnetic pavilion, 245;
new Observatory, 275;
future of, 283;
reflex zenith room, 304;
objects of, 316
Occultations by the moon, 212, et seq.
Octagon room, 125, 238, 242
Oldenburg, Mr., 30
Orion nebula, 268, 301
Parallax of stars, 303
Paramour, the, 65
Paris, conference at, 288
——, noon at, 151
Philip III., offer of, 19
Photographic registration, 244, 247, 252, 255;
refractors, 288
Photographs, star, 290
Photo-heliographs, 252, et seq., 279
Piazzi, discovery of, 222
Pleiades, the, 301
Polar plumes of the corona, 264
Polaris, 188
Pole-star, variation of, 184
Pond, John, sixth Astronomer Royal, his life, 97;
his reign, 98;
his salary, 98;
his assistants, 98;
his observations, 99;
censured by Visitors, 99;
his observations of stars, 303
Pound, James, 73
Precession of earth's axis, 184
Principia, publication of, 65
Proctor, R. A., attack of, 116
Ptolemy, Claudius, catalogue of, 185
Publication, the problem of, 48, 92
Quadrant, Heineken, 59
——, the iron, 73
Railway time, 152
Rain gauge, 238
Record rooms, 132
Reflection, observation by, 196
Reflex zenith room, 304
—— —— tube, 131
Refraction, effects of, 194
Right ascension, 186, et seq.
Roberts, Dr. Isaac, 301
Römer, discovery of, 78
Rosse, Lord, 268
Royal Society and Flamsteed, 46, et seq.
Saint-Pierre, Le Sieur de, proposal of, 23, 32
Sappho, 224
Saros of the moon, 67
Satellites, discovery of, 296
Saturn, atmosphere of, 279;
satellites of, 296
Schaeberle's comet, 280
Schedar, 184
Schiehallion, attraction of, 94
Schönfeld, 287
Scotchmen, anecdote of, 146
Sharp, Abraham, 46
Sheepshanks, Rev. James, on Airy, 107
Shuckburgh equatorial, 309
[320]Sidereal clock, 160
Sirius, 287
Sloane, Dr., 50
'Smith, Mr.,' his chronometer, 179
Solar photographs, 257
—— storms, 261, 282
Sound waves, 271
South, Sir James, 105, 114
South-east equatorial, the, 132, 221
Spectroscope, use of, 267
Spectroscopic Department, 266, et seq.
Spots, sun, 251, et seq., 281
Staff of Observatory, 137;
work of, 139, et seq.
Standard time, 21
Stars, observations of, 156, 176, 188;
origin of names of, 183;
movements of, 187;
catalogues of, 198, 284, et seq.;
composition of, 268, et seq.;
colour of, 271;
classes of, 287;
census of, 287;
photographs of, 288, et seq.;
motions of, 303, 315
Story, Mr. A. M., 97
Sun, distance of the, 74, 224;
spots on, 251, et seq., 281;
eclipses of, 263, et seq.;
chromosphere of, 268;
motions of, 315
Sunshine recorder, 238
Swiss time, 155
Tebb, Mr. W., 58
Tebbutt's comet, 280
Telescope, the great transit, 156
——, 28-inch, 275
——, astrographic, 289
——, Shuckburgh, 309
——, Thompson, 256, 279, 296
Thalèn, 268
Thermometer, use of, 192, 234
Thome, Dr., 287
Thompson photo-heliograph, 256, 279, 296
Time ball, 162
—— Department, the, 146, et seq.
—— desk, 161
——, foreign, 153
—— signals, 162
—— standard, 21
Transit, Halley's, 73
Transit circle, the, 114;
mode of observation with, 188, et seq.
Transit circle, Troughton's, 98
—— Department, 181, et seq.
—— observations, number of, 140
—— pavilion, 126, 175
—— room, 128, 147
Troughton's transit circle, 98
Uranus, discovery of, 217;
atmosphere of, 279;
satellites of, 296
Vanes, use of, 238
Venus, distance of, 223
Victoria, 224
Visitors, the Board of, 53;
censures Pond, 99;
work of, 106;
constitution of, 144
Visitors to Observatory, 175
Warrant for Flamsteed's salary, 39
Water telescope, 304
Weather predictions, 229, et seq.
Winds, study of, 237
Witt, Herr, discovery of, 223
Working Catalogue, the, 142
Zenith sector, 82, 305
—— tube, 75, 305
Zeta Ursæ Majoris, 306
Zubeneschamal, 184
LONDON: PRINTED BY WILLIAM CLOWES AND SONS, LIMITED,
STAMFORD STREET AND CHARING CROSS.
FOOTNOTES:
[1] Abraham Sharp had been with Flamsteed earlier than this—in 1684 and 1685.
[2] Sir Isaac Newton.
[3] The second circle was intended for the Cape Observatory, but Pond obtained leave to retain it. In 1851 it was transferred to the Observatory of Queen's College, Belfast.
[4] Mr. Thomas Lindsay, Transactions of the Astronomical and Physical Society of Toronto, 1899, p. 17.
[5] From Sir John Herschel's address to the British Association, September 10, 1846, thirteen days before Galle's first observation of the planet.